Degradex® Microspheres and Nanoparticles for Drug Delivery Research and Formulation Development
Bin Wu, PhD
Director, Technology and Product Development, and Theresa Logan, Project Manager, Phosphorex, Inc., Hopkinton, MA
Introduction
Microspheres and nanoparticles of biodegradable polymers such as PLGA, polylactic acid, and polycaprolactone have been widely used in drug delivery. Active pharmaceutical ingredients (APIs), whether small molecules or biologic compounds, may be encapsulated into microspheres and nanoparticles for specific drug delivery applications such as sustained release, targeted drug delivery, and protection of API from premature degradation. There are several drug-loaded PLGA microsphere formulations in the marketplace. For example, Lupron Depot® consists of leuprolide acetate encapsulated in PLGA microspheres and is used for the treatment of prostate cancer and endometriosis. It is subcutaneously administered at 1-month, 3-month, or 4-month intervals.
When drug-loaded PLGA microspheres are administered, the PLGA polymer starts to degrade in vivo by hydrolysis of the ester backbone into non-toxic products. As PLGA degrades, the drug molecules are gradually released from the microspheres. The drug release rate can be modulated by the selection of the type of PLGA polymer and adjusting the encapsulation process. For example, the following parameters can tune the drug release profile:
- The ratio of lactide to glycolide (L/G ratio) in the PLGA polymers; e.g., PLGA with an L/G ratio of 50:50 have the fastest drug release.
- The molecular weight or inherent viscosity of the PLGA polymer, where higher molecular weight provides slower drug release.
- The terminal group of the PLGA polymer, where carboxyl-terminated PLGA polymers offer faster drug release compared to ester-terminated PLGA.
Degradex® PLGA Particles
Degradex® PLGA microspheres and nanoparticles are polymeric particles in the size range of nanometers to microns. Both plain and fluorescently dyed particles are available. Plain PLGA microspheres and nanoparticles can be used in drug delivery research and formulation as control agents or can be used to test system compatibility before loading an API into the carrier. Fluorescent nanoparticles and microspheres can be used in imaging and diagnostic applications for the detection of binding events or signal enhancement. In addition, fluorescent particles can be used for fluid tracing, cell tracking, phagocytosis studies, fluorescence microscopy and drug discovery research.

Figure 1. Image of API-loaded PLGA microspheres
Degradex® PLGA Particles for Surface Conjugation
Degradex® PLGA particles may also be used as carriers in which drug molecules can be attached to the particle surface and delivered. The plain and fluorescent particles have surfaces functionalized with carboxyl groups, which allow for covalent attachment of proteins, peptides, antibodies, antigens, and small molecules. Drug conjugation to the Degradex® particles may be used to:
- Target a specific site, e.g. tumor1,2;
- Facilitate cell entry3;
- Help cross blood-brain barrier4;
- Increase solubility and bioavailability5;
- Extend plasma circulation time; typically this is done by using PEGylated PLGA nanoparticles6.
Handling and Use of Degradex® PLGA Particles
1. Reconstitution
Degradex® microspheres are normally packaged as lyophilized powder. Common applications often require the particles to be reconstituted in an aqueous medium such as DI water or a buffer. It is important to ensure that the particles are well suspended as a single particle suspension without any aggregation before use. The following is the recommended protocol for resuspending the Degradex® particles:
1. Remove the product container from freezer and allow to warm to ambient temperature.
2. Add DI water or buffer to the container.
3. Gently mix the contents in the container to ensure that the particles are well suspended; vortex and/or sonicate if necessary.
2. Particle Size Measurement
The particle size and size distribution of Degradex® PLGA particles can be measured with analytical methods including laser diffraction analysis, sedimentation, elutriation, microscope counting, Coulter counter, dynamic light scattering, and imaging particle analysis. Laser diffraction and Coulter counter are commonly used to measure the particle sizes of microspheres, whereas dynamic light scattering is the preferred method for nanoparticles.
For example, Figure 2 shows the particle size graph of a typical lot of Prod. 805122 using a Laser Diffraction Particle Size Analyzer. The particle size of Prod. 805211 is measured by a Dynamic Light Scattering instrument and an example of a typical graph is shown in Figure 3.
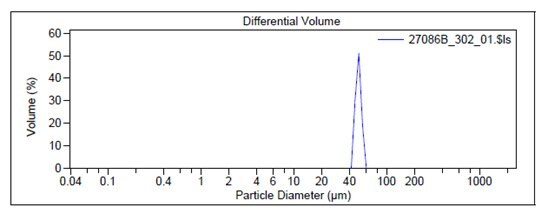
Figure 2. Particle size graphs measured by Laser Diffraction for Prod. 805122

Figure 3. Particle size graphs measured by Dynamic Light Scattering for Prod. 805211
3. Surface Conjugation
Degradex® PLGA particles contain carboxyl groups on the surface which can be used to react with amino groups on biologic compounds to form covalent bonds. The following conjugation protocol serves as a generalized guideline for conjugating biomolecules to Degradex® PLGA particles. It is expected that optimization will be required in order to achieve desired reactivity and stability.
- Weigh approximately 10 mg of carboxylated particles into a 1.5-2 mL polypropylene microcentrifuge tube. Add
0.2 mL 50mM MES buffer (pH 5.2), and vortex to suspend microspheres. Sonicate if necessary. - Prepare a 200 mg/mL EDC solution by dissolving 10 mg EDC (Prod. E1769) in 50μl 50mM MES
buffer (pH 5.2); Use immediately. - Add 20μl of the EDC solution to the microsphere suspension.
- Mix gently end-over-end.
- Add biomolecule equivalent to 200-500 μg. Mix gently end-over-end or briefly vortex.
- Incubate for 2-4 hours at room temperature with gentle mixing.
- Centrifuge mixture for 10 minutes at an appropriate G force. Note: Actual G forces needed to pelletize the
particles depend on the particle size. Save this supernatant for determination of the amount of bound biomolecule. - Resuspend microsphere pellet in 0.4 mL 10 mM Tris buffer containing 0.05% BSA (pH 8.0).
- Repeat Steps 7-8 and combine supernatants for use in bound biomolecule calculation.
- Store conjugated microspheres at 4 °C in Tris storage buffer. Conjugated particles should be used as soon as
possible due to the degradable nature of PLGA.
References
Az olvasás folytatásához jelentkezzen be vagy hozzon létre egy felhasználói fiókot.
Még nem rendelkezik fiókkal?