Polydiacetylene Nanotubes (PDNTs):
Nanotubes Based On Lipid Diacetylene Materials
Sang Beom Lee, Ph.D.
LIG Sciences, Inc. Export, PA 15632
1. Polydiacetylene Nanotubes (PDNTs): Chemistry, Structures and Properties
Polydiacetylene nanotubes (PDNTs) are self-assembled diacetylene nanotubes comprised of cross-linking of conjugated double and triple bonds. These PDNTs have remarkable properties which include uniform dimensional structures and responsive chromic characteristics that can be utilized as is, within a solution, or self-assembled onto various substrates, such as silica, polymer, and metal surfaces. PDNTs have been of great interest to chemists and biologists from universities, pharmaceutical companies, paint/coating companies, and opto/electronic companies in need of cheap, pure, predictable and well-defined lipids and chromatic nanotubes.
Polydiacetylenes (PDA)
Polydiacetylenes (PDA) are conjugated polymers produced by UV (254 nm) free radical polymerization of lipid diacetylene monomers (Figure 1) that have self-assembling property, and their microstructures were first reported by Yager et al. in 1984.1 For polymerization, diacetylene monomers need to be well aligned, which is achieved by molecular self-assembly of the diacetylene monomers. The self-assembly process often generates interesting nanostructures, such as nano- and micro- tubes and micelles of lipidic diacetylenes.2-8 Various attempts have been made to control diameters and lengths of nanotubes by changing chemical modifications and fabrication methods of amphiphilic diacetylene lipids. Fabricating well-defined diacetylene nanotubes in high purity has been of a key interest among scientists who are studying biosensors, microelectronics, and drug delivery system (DDS).

Figure 1.Photopolymerization of diacetylene lipid monomer.
PDA undergoes a blue-to-red color transition when they are exposed to a stimulus, such as heat, chemical exposure, extreme pH, or mechanical stress. In their ground state, polymerized diacetylenes absorb light at 650 nm, making them appear blue. When exposed to a stimulus, the absorption shifts to 550 nm, due to shortening of the p-conjugated bonds in the polymer backbone, making them appear red. Color transition of polydiacetylenes has been used for developing biosensors by introducing specific ligands to the monomers, so that the ligands can bind to the receptors, such as viruses and bacteria (Figure 2).9-12

Figure 2.Color transition mechanism of diocetylenic polymer by ligand-receptor binding.
Polydiacetylene Nanotubes (PDNTs)
One of the promising diacetylene-based synthetic nanostructures for various real world applications is the nanotube structure derived from suspensions of diacetylenic phosphatydlcholines.13 When solutions of these molecules are heated and cooled below the melting temperature of lipid, bilayer sheets form and roll up into a tubular formation with an overall helical structure (Figure 3). Almost all of the nano-structure forming lipids have been chiral molecules.14-18 Most derivatives of acetylcholine form spherical liposomes, but there have been discovered molecules that can form the lipid tubules, which display some interesting properties. While there appears to be some evidence that chirality of the monomer lipid is strongly favored as a requirement for nano-structure formation, it is not an absolute necessary requirement.6 An achiral diynoic phosphatidylcholine was shown to produce helical twisted ribbon structures in which the handedness of each ribbon was constant where there were equal numbers of each type of optical isomer utilized in the preparation.19,20 Tubules formed from another achiral phosphatydlcholines were equally divided between left and right handed helices but the chirality of the whole preparation could be altered by spiking the mixture with a small amount of a chiral derivative. This observation has led to the conclusion that the organization of these structures is more influenced by the diynes in the hydrocarbon tails than the intrinsic chirality of the molecule.19 It should be noted that in most cases the structures formed by achiral diacetylenyl lipids were not homogeneous preparations of nanotubes.
In addition to chirality, there seems to be a strong preference for molecules with two lipid diacetylene chains. In light of this, it is interesting to note that a very simple single chain achiral diacetylene has been developed, contrary to the current way of thinking, that can self- assemble into a remarkably homogeneous population of nanotubes and can be induced to form other nano structures (Figure 3 and 4).

Figure 3.Synthesis and molecular self-assembly of a single chain diacetylene monomer and polydiacetylene nanotubes (PDNT).

Figure 4.LIG Sciences' nomenclature for their diacetylene monomers is based on the number of carbons in each section, including the hydrophic tail and hydrophilic head groups.
Lee et al.7,8 have described the nanotube forming ability of diacetylene monomers (Figure 4), which are achiral amphiphilic diacetylenes with secondary amine salt head groups to form uniform self-assembled nanotubes. The diacetylenic monomers are composed of two different parts that contribute to the interesting assembly of microstructures while in an aqueous media. One is hydrophobic tail and the other one is hydrophilic head group. Both parts play important roles when they are self-assembled into microstructures, such as liposomes and nanotubes.
Figures 5 and 6 are SEM images of nanostructures formed from DM-12-8-2-2-Br, which were found to be extremely pure and homogenous polydiacetylene nanotubes (PDNT) with an inner diameter (I.D.) of 34 nm, an outer diameter (O.D.) of 98 nm and length (L) of about 1 micron.

Figure 5.SEM image showing uniform diameter and purity of PDNT.

Figure 6.SEM image showing uniform nanotube openings of PDNT.
These linear nanotubes have been found to be composed of five bilayers (Figure 7) are seamless when observed by SEM and TEM. Utilizing specific preparation conditions, it has been found that unique nanotube morphologies, such as the branched nanotubes shown in Figure 8, can be created. This shows that specific nanostructures and their morphologies can be created not only by altering the chemical structures of lipid monomers but also by changing the self-assembly process.

Figure 7.Polydiacetylene nanotubes before and after UV-polymerization.

Figure 8.SEM image of branched polydiacetylene nanotubes.
PDNTs are polymerized by crosslinking at alkyne bond sites by exposure to ultraviolet light (Figure 7). After crosslinking, the nanotubes undergo a blue-to-red color transition when they are exposed to various types of stimuli, such as heat or mechanical stress. A color change from blue to red occurs around 70 oC due to the twisting of the polymer backbone when exposure to heat8. In most cases, this color changing is reversible, becoming blue again when the temperature returns below room temperature. One exception, as shown in Figure 9, is when the nanotubes are suspended in water, where the color change back to blue does not occur when the temperature goes back to room temperature. This color change from blue to red is also observed from applying stress on the polymerized nanostructure. It shows that these polymerized self-assembled structures have a potential to be used in sensors to detect the stress caused by enzymes, encapsulants or adsorbants that are themselves the species being sensed or that create the stress on the nanotube after coming in contact with the species being sensed. Specifically a nanotube with adsorbed/absorbed proteins may impart stress on the nanotube through protein conformational changes due to an environment change, thus creating a color change.

Another interesting result is that PDNT can be uniformly dispersed in polystyrene solution. The PDNT containing polystyrene solution was blue, indicating that the tube structures were not altered by incorporation into the polymer. By heating to 70 oC the polymer turned red (Figure 10) and cooling made the material back to blue, reversibly. At 110 oC, conversely, the polymer changed from red to clear, however, did not reversibly return to red when cooled below 110 oC. These reversible, irreversible chromatic changes can be utilized for practical applications, such as paints that reversibly change colors to warn of hot surfaces or that irreversible change colors for items such as frozen food or other perishables that may experience an undesired warming in transit to a store.

Figure 10.Color transition of polydiacetylene nanotubes in polystyrene.
The reversible color change by heating up to 70 oC is also observed from the PDNT containing polyurethane elastomer. Interestingly when the elastomer is stretched, it loses its blue color, becoming red, then returns to blue upon the removal of the stress and relaxing it to the original condition. It indicates that the incorporation of non-modified PDNTs into polymers have potentials to yield smart chromatic materials and coatings that are able to monitor the changes in mechanical stress experienced on surfaces.
Figure 11 shows the color changes of PDA in an aqueous environment before/after irradiation of light and the effect of various additives on the color of the polymerized PDA. Unpolymerized or uncrosslinked PDNT (tube 1) in water was a slightly turbid white solution which turned blue after cross-linking (tube 2) by exposing to UV radiation. Addition of bacteria (1 X 109 cells) to cross-linked PDNT caused a flocculation of the suspension within 30 minutes (tubes 3 and 4). Note that all of the colored material was precipitated by the bacteria. Addition of SDS results in the color change to yellow (tube 5) which is unusual as most polydiacetylenes show only a blue to red color change. Other surfactants showed various results; CTAB had no effect (tube 6) while CTAC, which differs only in the counter ion (chloride verses bromide), caused the classic blue to red conversion (tube 7). Pluronic had no effect but exposure to triton-X100 made a purple color (tubes 8 and 9, respectively). Finally, the effect of salt concentration (by addition of NaCl, tube 10) or pH (by addition of HCl or NaOH) on the color change has not been observed. This broad variety of color changes for the different reactions that was found with PDNT is unusual. It is anticipated that this unique feature of PDA can be exploited in the design of colorimetric biosensors that utilize PDNTs that are composed of PDA.
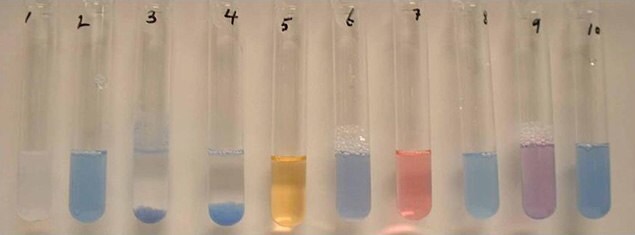
Figure 11.Effect of various additions of polymerized PDA. Tube: 1) Non-polymerized PDA; 2) Polymerized PDA; 3) E. Coli.; 4) B. subtilis; 5) sodium dodecyl sulfate (SDS); 6) cetyltrimethylammonium bromide (CTAB); 7) cetyltrimethylammonium chloride (CTAC); 8) Pluronic; 9) Triton-X 100; 10) NaCl.
2. Features and Potential Applications of PDNTs
The mechano chromism property of PDNTs can be utilized in future products. Any mechanical stress will induce microstructural changes in the nanotube/polymer composite coatings, resulting in the color changes. This color changing paint could be utilized in situations where unwanted yielding to mechanical stress needs to be monitored and detected such as in the aluminum skins of aircraft, building components, critical bridge members, etc. In addition, the thermo chromism properties can also be utilized in these nanotube/polymer composite coatings to provide visual temperature warnings or indications of hot surfaces which are painted with this composite coating. Also, there is a potential to utilize PDNTs as a support platform of enzyme delivery to facilitate biochemical and biological reactions. This platform could be used in biosensors where enzymes tailored for interacting with specific chemical or biological materials might create stresses on the nanotube resulting in a color change. It could be utilized in a microassay card for onsite identification of unknown substances whether it is narcotics, pollutants, biological/chemical warfare agents, etc. Table 1 summarizes salient features of the PDNTs.
3. Polydiacetylene Nanotubes (PDNTs) available
Listed in Table 2 are the high-purity PDNTs (773484 and 773492) offered in our catalog. These PDNTs are extremely pure and well-polymerized due to the excellent alignment after the self-assembly of diacetylene monomers. SEM images of PDNT-12-8-2-2-Br (773484) in Figures 5 and 6 show the purity and nanotube lumen openings.
References
To continue reading please sign in or create an account.
Don't Have An Account?