Chemical Synthesis of Monodisperse Magnetic Nanoparticles
Sheng Peng, Shouheng Sun
Department of Chemistry Brown University Providence, Rhode Island 02912, USA
Material Matters 2009, 4.1, 14
Introduction
Magnetic nanoparticles have attracted tremendous attention due to their novel properties and their potential applications in magnetic recording, magnetic energy storage and biomedicine.1-3 Thanks to numerous research efforts, great progress has been made on the design and fabrication of magnetic nanoparticles with controllable size, morphology, structure, composition, and magnetic properties. A variety of “bottom-up” synthesis methods have been developed and, as a result, magnetic nanoparticles are now routinely made from solution-phase thermal decomposition, metal reduction, and metal salt co-precipitation. To produce monodisperse nanoparticles with a standard deviation in diameter of less than 10%, a burst nucleation event should occur in a supersaturated solution without the formation of new nuclei and the produced nuclei would grow uniformly thereafter. Practically, a hot-injection procedure is often employed to achieve the burst nucleation by rapid introduction of reagents into the reaction vessel. Alternatively, a heating strategy can be adopted, in which a reaction mixture is prepared at low temperature followed by heating to initiate the particle nucleation and growth. In this paper, we summarize briefly the recent progress in synthesizing monodispere, magnetic nanoparticles.
Magnetic Ferrite MFe2O4 (M = Fe, Co, Mn, etc) Nanoparticles
Magnetic ferrites, which adopt cubic spinel structures and have a general formula of MFe2O4, are one of the most important classes of magnetic materials. In such ferrites, oxygen atoms exhibit face-centered cubic (fcc) close packing with M2+ and Fe3+ cations occupying either tetrahedral or octahedral interstices. Depending on the identity of the M2+ in the lattice, different ferrites can show distinctive magnetic properties.
Recently, a facile organic phase synthesis utilizing high temperature reaction of metal acetylacetonates and 1,2-alkanediols was developed.4 For example, monodisperse 6 nm single-crystal Fe3O4 nanoparticles (Figure 1a) were synthesized by heating (300°C) a reaction mixture of Fe(acac)3 (Product No. 413402), 1,2-hexadecanediol (Product No. 213748), oleic acid (OA) (Product No. O1008), oleylamine (OAm) (Product No. O7805) and benzyl ether (Product No. 108014). The particle size could be tuned to 20 nm by seed-mediated growth using small particles as seeds. The recipe was readily extended to the synthesis of other ferrite (MFe2O4, M = Co, Mn, Ni, etc) particles by incorporating a stoichiometric amount of M(acac)2 together with Fe(acac)3 into the reaction mixture. The control of magnetic properties of different MFe2O4 is better illustrated in the 16 nm nanoparticles of Fe3O4 and CoFe2O4. The Fe3O4 particles are superparamagnetic at room temperature with a saturation magnetization (Ms) of 83 emu/g, close to the bulk value of magnetite. At lower temperature, the particles become ferromagnetic with coercivity (Hc) of 450 Oe at 10K. In comparison, the 16 nm CoFe2O4 particles (Figure 1b) exhibit a room temperature Hc of 400 Oe and a much larger Hc of 20 kOe at 10 K (Figure 1c).
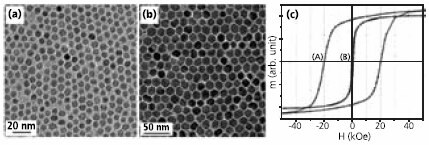
Figure 1.Transmission electron microscopy (TEM) images of (a) 6 nm Fe3O4 particles and (b) 16 nm CoFe2O4 particles; (c) Hysteresis loops of 16 nm CoFe2O4 particle assembly measured at A) 10 K and B) 300 K. Reproduced from Ref. 4 with permission from the American Chemical Society.
Fe3O4 nanoparticles can also be made by high temperature decomposition of iron(0) pentacarbonyl Fe(CO)5 (Product No. 481718) in presence of OA followed by oxidation using trimethylamine N-oxide (CH3)3NO (Product No. 317594) or air,5 or decomposition of metal-oleate complexes.6 Cube-like MnFe2O4 particles are synthesized by controlling the surfactant/precursor ratios.7 Ultra-small Fe3O4 nanoparticles are produced by thermal decomposition of Fe(CO)5 in the presence of methyl catechol.8 Hollow Fe3O4 particles were fabricated by controlled oxidation of Fe/Fe3O4 particles utilizing the nanoscale Kirkendall effect.9
Metallic Fe and Co Nanoparticles
Metallic Fe (Product No. 637106, 544884) and Co (Product No. 697745) nanoparticles are important for various magnetic applications due to their high magnetization values (Ms: 218 emu/g for Fe and 162 emu/g for Co). However, fabrication of high moment Fe and Co nanoparticles has been very challenging due to their chemical instability. Recent syntheses prove that it is now possible to stabilize these reactive nanoparticles for high moment magnetic applications.
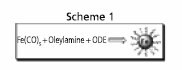
Scheme 1.Schematic outline of a facile synthesis of Fe nanoparticles via thermolysis of Fe(CO)5 in 1-octadecene (ODE). Reprinted from Ref. 10 with permission from the American Chemical Society.
The most common approach for fabricating monodisperse Fe and Co nanoparticles is via thermal decomposition of the corresponding organometallic precursors, particularly the carbonyls. Recently, we reported a facile one-step synthesis (Scheme 1) of monodisperse Fe nanoparticles (s < 7%) by thermolysis of Fe(CO)5 in 1-octadecene (ODE) (Product No. O806) with OAm.10 The primitive Fe particles were readily oxidized during post-synthesis treatment in air, giving 8 nm/2.5 nm Fe/Fe3O4 nanoparticles (Figure 2a), with both the core and shell being amorphous. As-synthesized Fe/Fe3O4 particles are superparamagnetic at room temperature with Ms of ~67 emu/g. The value is normalized as 103 emu/g (Fe+Fe3O4) after surfactant removal and is close to the sum of the core- and the shell-contributions. Further oxidation of the particle dispersion in air led to rapid magnetic moment decline and severe particle agglomeration (Figure. 2c). However, once a dense shell of crystalline Fe3O4 was created over the Fe core via controlled oxidation using (CH3)3NO, Fe in Fe/Fe3O4 nanoparticles was more efficiently stabilized. The resulting core/shell nanoparticles of Fe/Fe3O4 (5nm/5nm) (Figure 2b) show an initial Ms of ~62 emu/g particles. Compared to the untreated particles, the moment of the oxidized particles drops much slower and is stabilized at 56 emu/g after 24 h exposure to air (Figure 2c). This crystalline Fe3O4 protection strategy has proved to be effective in stabilizing metallic Fe and giving stable core/shell nanoparticles of Fe/Fe3O4. Similarly, Co particles can be protected by a crystalline Fe3O4 shell as well.11

Figure 2.TEM images of (a) as-synthesized 8 nm/2.5 nm Fe/Fe3O4 nanoparticles, bar scale 100 nm (inset: higher resolution, bar scale 10 nm); (b) control-oxidized 5 nm/5 nm Fe/Fe3O4 particles, bar scale 10 nm. (c) The magnetic moment change of Fe/Fe3O4 core/shell particles versus time when exposed to air at room temperature. Reproduced from Ref. 10 with permission from the American Chemical Society.
Recent synthetic efforts have led to numerous other methods for making monodisperse Fe or Co nanoparticles. For example, 7 nm Fe nanocubes were made by thermolysis of (Fe[N(SiMe3)2]2).12 Hollow Fe nanoframes were formed via molten salt corrosion.13 Co particles with various crystal phases were synthesized by either cobalt carbonyl decomposition or cobalt salt reduction.14 Co nanorods were obtained by decomposition of [Co(η3-C8H13) (η4-C8H12)].15 Hollow Co particles were produced by simultaneous fast out-diffusion of CoO (Product No. 529443) oxide species and surface reduction of the oxides by OAm.16
Magnetic Alloy Nanoparticles: FePt and FeCo
Magnetic iron-platinum (FePt) alloy nanoparticles made by solutionphase chemical synthesis have shown great potential for high performance permanent magnets and for high density data storage applications.1 Their magnetic properties can be tuned not only by particle size, but also by their composition and structure. fcc-FePt nanoparticles have been fabricated by simultaneous decomposition of Fe(CO)5 and reduction of Pt(acac)2 (Product No. 523038).17 The synthesis, annealing and alignment of shape-controlled FePt nanocubes and nanorods were also reported.17,18
Very recently, dispersible fct-FePt particles were obtained via thermal annealing of MgO-coated fcc-FePt particles, followed by MgO removal.19 First, MgO (Product No. 529699) was coated over the fcc-FePt surface via decomposition of Mg(acac)2 (Product No. 129577). The fcc-FePt/MgO nanoparticles were annealed to 800°C. The MgO shell was then removed via dilute acid washing and the fct-FePt particles were stabilized in hexane with a mixture of OA and 1-hexadecanethiol (Product No. 674516). The coercivity of the fct-FePt particles reaches 2 T at 5K and 1 T at 300 K. The TEM images of the nanoparticles after each stage are shown in Figures 3a thru 3c.

Figure 3.TEM images of: (a) as-prepared fcc-FePt particles (bar 20 nm), (b) MgO-coated and annealed FePt (bar 20 nm), (c) dispersible fct-FePt particles after shell removal and surface modification, reproduced from Ref. 19 with permission from Wiley-VCH, (d) 15 nm FeCo particles, reproduced from Ref. 20 with permission from the Nature Publishing Group, (e) 20 nm FeCo, reproduced from Ref. 21 with permission from the American Chemical Society, and (f) 7 nm FeCo particles, inset: HRTEM showing graphitic shell (bar 2nm), reproduced from Ref. 22 with permission from the Nature Publishing Group.
FeCo alloys have the highest magnetic moment under ambient conditions, reaching 245 emu/g. Uniform 15 nm FeCo alloy particles (Figure 3d) (Ms 160~180emu/g) were synthesized by co-decomposition of Fe(CO)5 and Co(N(SiMe3)2)2.20 20 nm FeCo particles (Figure 3e) (Ms 207emu/g) were made by polyol co-reduction of Fe(III)- and Co(II)-acetylacetonates (Prod. Nos. 517003 and 227129) in OA/OAm under a reductive (Ar+7%H2) atmosphere.21 Air-stable 7 nm FeCo particles (Figure 3f) with a graphitic shell were prepared via chemical vapor deposition, followed by surface modification using phospholipidpoly( ethylene glycol).22
Rare-earth Metal Based Hard Nanomagnets of SmCo5 and Nd2Fe14B
Rare-earth hard magnets, composed of rare-earth elements and 3d transition metals, are technically important permanent magnets. Two rare-earth systems, SmCo and NdFeB, have been at the center of hard magnetic research due to the need for nanocomposite magnets with enhanced coercivity and remnant magnetization for high performance permanent magnet applications. Traditional physical fabrication techniques, including melt spinning and ball milling, are often used to produce granular hard magnetic materials, but provide only limited control over the size of the final magnetic grains. Solution-phase chemical syntheses that have proven to be successful in preparing monodisperse magnetic nanoparticles were recently studied extensively for making nanostructured SmCo and NdFeB magnets.
Polyvinyl pyrrolidone (PVP) assisted co-reduction of Sm- and Co-nitrates in tetraethyleneglycol (TEG) (Product No. 110175) were used to make the SmCo-magnets.23 The resulting air-stable 10×100 nm blade-like rods (Figure 4a) were primarily hexagonal SmCo5 with slight Sm2Co17 content. The intrinsic coercivity and magnetization of the particles were 6.1 kOe and 40 emu/g at room temperature, and 8.5 kOe and 44 emu/g at 10 K, respectively (Figure 4b). Nanocrystalline SmCo5 (Product No. 692859) hard magnets were also prepared by reacting core/shell structured Co/Sm2O3 particles (Co/Sm atomic ratio 4.3:1) with metallic Ca (Product No. 215147) at 900 °C to reduce Sm2O3 and to promote interfacial diffusion between Sm and Co.24 KCl (Product No. 409316) was used as the dispersion medium to promote the reduction at relatively low temperatures and to prevent the sintering of SmCo5into large single-crystals. XRD confirmed the hexagonal SmCo5 structure in the annealed product, while HRTEM revealed nanocrystalline grains (Figure 4a). The coercivity reached 24 kOe at 100 K and 8 kOe at room temperature with remnant moments of 40-50 emu/g (Figure 4b). The solution phase reaction and high temperature reduction process was extended to the synthesis of exchange-coupled SmCo5/Fex nanocrystalline composites with a maximum coercivity of 11.6 kOe and remnant moment reaching 90emu/g at room temperature (Figures 4c and 4d).25
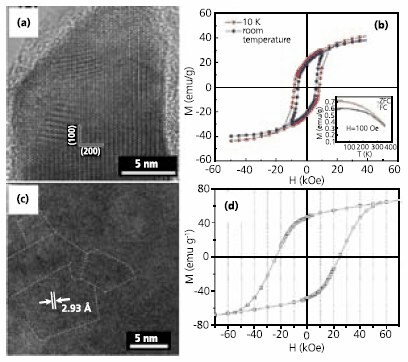
Figure 4.SmCo5 magnets made from the PVP process: (a) HRTEM image (b) ambient and low temperature (10K) hysteresis loops, reproduced from Ref. 23 with permission from the American Institute of Physics. Nanocrystalline SmCo5 via reductive annealing of Co/Sm2O3: (c) HRTEM image (d) 100 K magnetic measurement, reproduced from Ref. 24 with permission from Wiley-VCH.
Conclusion
A burst in the research efforts via chemical synthesis has led to the production of various monodisperse magnetic nanoparticles with controlled magnetic properties and chemical stability. In this short review, we have summarized recent exciting developments in some well-known magnetic nanoparticles of ferrites, metals, alloys and rare-earth magnets. These magnetic nanoparicles can be superparamagnetic (Hc = 0) or strongly ferromagnetic (Hc > 1 T) with magnetic moments ranging from those at the ferrite level of ~80 emu/g to the highest alloy value of over 200 emu/g. These magnetic nanoparticles have been studied extensively for various applications. For example, hard magnetic nanoparticles with large coercivity are promising for future ultra-high density information storage applications; soft magnetic nanoparticles with low coercivity and high magnetic moment are key components for electromagnetic devices. Composite nanoparticles containing exchange-coupled magnetically hard and soft phases are predicted to be the materials of choice for future permanent magnets with optimum energy product. Superparamagnetic nanoparticles have shown great potential for magnetic resonance imaging contrast enhancement and for magnetic fluid hyperthermia.
Acknowledgement
The magnetic research was supported by ONR/MURI N00014-05- 1-0497 and DARPA/ARO W911NF-08-1-0249.
References
如要继续阅读,请登录或创建帐户。
暂无帐户?