Gold Nanorod Synthesis
Brantley C. Henson, Justin T. Harris, Kimberly A. Homan
NanoHybrids Inc.
Material Matters, 2017, 12.1
Introduction
Plasmonic nanoparticles have unique optical properties that can be tailored to suit a variety of applications in the biotechnology1–8 and electronics9–16 industries. Gold nanorods (Figure 1), a particular subset of plasmonic nanoparticles, are of interest for biomedical applications due to their tunability in the nearinfrared (NIR) light spectrum and biological inertness.17–20 There are numerous techniques for the synthesis of gold nanorods, including templating,21–25 electrochemical growth,26–28 and reverse micellar systems.29–30 Here, we focus on seed-mediated colloidal growth methods. While there are multiple published variations on the seed-mediated colloidal growth of gold nanorods,29,31–38 our discussion will examine the well-known approaches pioneered by Nikoobakht and Murphy.31,39 Seed-mediated colloidal growth has many advantages over the aforementioned methods,41 including no need for specialized equipment and straightforward scalability—eventual requirements for clinical translation.43–44 Although these methods are well understood, their sensitivity to small changes in reaction conditions can lead to a lack of control over the size, shape, purity, and aspect ratio of rods from batch to batch.19,33,36–37,44–50 In this review, we briefly discuss seedmediated gold nanorod synthesis, and then present a detailed discussion of the common pitfalls and how best to avoid them.

Figure 1.TEM image of typical gold nanorods.
Synthesis
Basic synthesis follows the work of Nikoobakht and Murphy,31,37,39,51–52 where precise reactant concentrations and volumes are discussed. Briefly, a growth solution composed of cetyltrimethylammonium bromide (CTAB, Prod. No. 52370), water, silver nitrate (AgNO3, Prod. No. 792276), and chloroauric acid (HAuCl4, Prod. No. 254169) is prepared. If a multisurfactant system is required for longer aspect ratios, benzyldimethyl ammonium chloride (BDAC) is also typically included, though other surfactants such as sodium oleate (Prod. No. O7501) have also been reported.46,53 Ascorbic acid (Prod. No. A92902) is then added to reduce the Au3+ to Au1+. To template further reduction of gold, small gold nanoparticle seeds are then added to the solution. The seeds are synthesized via a rapid reduction of HAuCl4 by sodium borohydride (Prod. No. 480886) in a CTAB or citrate solution. Upon addition of the seeds, Au1+ is further reduced to metallic Au0, with the surfactants and Ag serving to break the symmetry through preferential deposition on the {110} facets of gold, inhibiting growth54 and encouraging growth parallel to the {001} plane, resulting in anisotropic gold nanorods.31
Six Common Pitfalls in Gold Nanorod Synthesis
The Importance of Good Synthesis Preparation (1)
Slight adjustments in overall concentrations, impurities, and nucleation sites can result in significant differences in particle size, shape, and purity, rendering reproducibility a concern. Thus, successful and repeatable gold nanorod synthesis is highly reliant upon proper and consistent preparation and lab technique. First, to ensure even growth, nucleation sites must be well controlled. As a general requirement for most metallic nanoparticle syntheses, all glassware and stir bars must be thoroughly cleaned with aqua regia55 to remove residual metals. Any residual metal will lead to unwanted pre-nucleation in the growth solution, making controlled synthesis impossible. All water used throughout the synthesis process, including glassware cleaning, needs to be 18.2 MΩ・cm ASTM Type I water (commonly referred to as deionized ultrafiltrated, DIUF, or “Nanopure™” water). Likewise, the lab space should be clean, with minimal dust.
Another common cause of variability from lab to lab, or even person to person, is the reagent preparation; even when buying from the same supplier, minor changes in impurities from lot to lot can affect the final product of the nanorod synthesis. For example, Korgel et al. report on the effect of the CTAB source on nanorod synthesis.33 Interestingly, the results indicate trace impurities in CTAB, particularly iodine, are actually required in order to produce high quality rods.56 Similarly, NanoHybrids has found changing the gold acid source can affect the overall size and longitudinal surface plasmon resonance (LSPR) of the resulting rods. For these reasons, documentation and tracking of reagents is necessary for all synthesis. Whenever changing reagent lots or sources, pilot reactions should first be performed to set the new baseline for further size and shape tuning. Other considerations include reagent age and storage conditions. For ease of reaction and to minimize inherent weighing errors, many groups choose to make stock solutions of reactants. Recent work by Murphy et al.38 demonstrated solutions of silver nitrate and ascorbic acid are best prepared fresh due to their susceptibility to photodegradation. Likewise, the age of CTAB solutions has been shown to affect the final product.38 Depending on the quantity and scale of reactions a lab produces, these effects can either be detrimental or potentially leveraged for increased control over reaction kinetics. Again, proper documentation is paramount to success.
Aggregation Happens: Rod Instability (2)
One of the most common problems during synthesis is nanoparticle aggregation. Knowing when aggregation occurs is just as important as knowing how to prevent it. Aggregation of nanorods with an absorbance in the NIR spectrum can be easily detected by ultraviolet-to-visible (UV/Vis) spectrophotometry. The main indication of aggregation is the presence of a “shoulder” in the extinction spectra. A typical “good” spectrum is shown in Figure 2A. Note the high ratio of the LSPR peak to the transverse surface plasmon resonance (TSPR) peak (labeled as L/T, Figure 2A), and the narrow, symmetric shape of the LSPR peak. Figure 2B shows the result of slight aggregation. Note the obvious lack of symmetry and right-side skew of the LSPR band caused by plasmon coupling in gold nanorod aggregates. Similarly, Figure 2C shows particles made with a dual surfactant system (CTAB + BDAC). While not as obvious as Figure 2B, the asymmetry of the LSPR band and increased full width half maximum (FWHM) indicates problems with the synthesis, such as high polydispersity of size and aspect ratio or slight aggregation. These problems are not inherent to all dual-surfactant reactions and, thus, is an indicator of a failed reaction. Also, note the shape of the TSPR band (BDAC Trans Peak) in Figure 2C. The additional small shoulder is due to subtle differences in the shape of the rods themselves, along with the associated impurities (cubes vs. spheres). Even in a “good” dual-surfactant reaction with a narrow LSPR and high symmetry, this variation in the TSPR band is expected. A spectrum of nanorods with high amounts of shape impurities (large numbers of spheres and cubes) is shown in Figure 2D and discussed further in pitfall #4. Finally, severe aggregation can be easily identified by particle precipitation or a change in appearance from various shades of red to dark gray or purple, such as in Figure 2E.

Figure 2.Spotting nanorod aggregation and shape impurities using normalized UV/Vis spectra. A) Ideal spectra of gold nanorods synthesized with a single surfactant (CTAB) is evidenced by a high LSPR/TSPR peak ratio (L/T) and a narrow symmetric LSPR peak. B) Spectra displaying aggregates in a “shoulder.” C) Spectra of particles synthesized in a binary surfactant (CTAB and BDAC) is shown by the shape of the transverse peak. The spectrum contains a wide asymmetric LSPR peak indicating wide size distribution. D) Spectra of particles exhibiting high amounts of shape impurities. E) Photo of (1) as-synthesized and (2) aggregated gold nanorods.
The root cause of aggregation is often difficult to identify due to the complexity of the synthesis. Unexpected impurities in new reagents, reagent aging, incompatible reagent combinations, contamination, and improper product purification (discussed later) are all potential sources of the problem. To mitigate these problems and maintain general synthesis consistency, it is recommended that regular small-scale pilot studies (20–30 mL reactions) be performed on a monthly to quarterly basis to verify the quality of reagents and identify sources of contamination or degradation. It is important to note that while small-scale reactions are used for verification purposes and reproducibility in comparison to previous studies, the exact peak absorption typically shifts when the reaction is conducted at a larger scale; specifically, a lower LSPR is expected upon scale-up. Moreover, the actual resultant peak is also dependent upon vessel size, shape, spin speed, and heating efficiency. As a result, the expected LSPR shift should be determined for any new glassware and protocol for consistent targeting of LSPR wavelengths.
Poor Control Over Aspect Ratio (3)
One of the unique properties of gold nanorods is the tunability of their optical peak absorption from the visible to IR range simply by changing their aspect ratio (defined as the ratio between length and width/diameter of the nanorod). Within the standard seed-mediated growth protocols, this requires breaking the symmetrical growth, which is thermodynamically favored, to encourage growth along a single axis. To this end, silver nitrate is a reliable tool for lengthening gold nanorods.31 As seen in Figure 3A, generally increasing the silver nitrate concentration increases the aspect ratio and corresponding LSPR, but this method only works up to ~850 nm. For aspect ratios greater than 4.5 or an LSPR greater than 850 nm, Nikoobahkt demonstrated that varying the ratio of BDAC and CTAB in a binary surfactant system yields aspect ratios up to 10 (Figure 3B).31 Values as high as 70 have been reported by implementing a multistage addition of growth solution to seeds, as reported by Murphy et al.57 Perez–Marzan demonstrated a correlation between increased seed amount and decreased aspect ratio.58 Whichever approach is chosen, the delicate interplay within the multivariable synthetic system illustrates the importance of tight control over the reactant concentrations while making it possible to synthesize a wide range of high quality gold nanorods (Figure 3D). For consistency, massing your reactants versus relying on volumetric techniques is recommended.
It is also worth considering that in all syntheses involving gold nanorods, there is a tendency for the nanorod aspect ratio to decrease over time, which will cause a blue-shift in the LSPR peak. This blue-shift is thought to be a consequence of Ostwald ripening, via a softening of any sharp edges to a more thermodynamically stable arrangement.42 You can, therefore, artificially speed up the rate of Ostwald ripening by thermal treatment of the particles in an aqueous solution, lowering their aspect ratio (Figure 3C).

Figure 3.Controlling the aspect ratio of gold nanorods. A) Effect of increasing silver nitrate concentration on the nanorod aspect ratio and resulting optical properties upon synthesis. (1–5) indicate increasing additions of 4 mM AgNO3 from 50–300 μL to identical growth solutions. B) The effect of using single (1) and binary (2) surfactant systems in the synthesis of gold nanorods. C) The effect of heating on the LSPR peak (closed dots) and TSPR peak (open dots) of aqueous solutions of gold nanorods. D) An array of nanorods synthesized using combinations of tuning reactions discussed.
Poor Shape Control (4)
During any nanorod synthesis, the appearance of shape impurities is inevitable. However, these impurities can be monitored and minimized. Figure 2D illustrates a nanorod reaction with poor shape purity. Unwanted spheres in the reaction have a strong absorbance at 510–525 nm, resulting in an increase in the apparent TSPR peak. Thus, monitoring the LSPR/TSPR ratio is a strong indicator of overall shape purity. A “good” ratio of at least 3.5 indicates ~90% shape purity.59–60 If a high quantity of impurities is observed, this can indicate that reactants are too old, not enough AgNO3 was used in the reaction, reaction temperature was too high, or too high of a BDAC/CTAB ratio was used, to name a few potential problems. Post-synthesis separations can be performed to remove unwanted shapes, as discussed in the next section. Likewise, several variations of the basic cylindrical shape of nanorods can emerge during their synthesis. Binary surfactant reactions such as those using both BDAC and CTAB generate rods with sharper corners, giving them a more “brick-like” structure (Figure 4A) versus the standard CTAB reaction (Figure 4B). Spectrally, this can be observed as an additional small peak slightly red-shifted from the typical TSPR peak (Figure 2A vs 2C). “Dog bones” or “dumbbells” are another feature that can dramatically affect the spectra of nanorods (Figure 4C). Several groups report these features appear upon secondary additions of ascorbic acid and are pH driven.32,50–51 We have observed these same features via adding excessive ascorbic acid in a single addition. It is possible to “soften” features like sharp corners and dog bones through thermal treatment, resulting in a reduced intensity of their additional spectral features and an overall blue-shift of their LSPR.

Figure 4.TEMs of gold nanorods demonstrating a correlation between absorbance spectra and anisotropic geometry of gold nanorods. Insets are typical corresponding UV/Vis spectra for the given shape, spanning 400–1,000 nm. A) Gold nanorods synthesized in binary surfactant system containing CTAB and BDAC. B) Gold nanorods synthesized using only CTAB. C) Dog bone- or dumbbell-shaped nanoparticles.
Insufficient Purification (5)
After any synthesis, purification is required to remove excess reactants and reduce the overall concentration of CTAB for storage. If left in the original reactants for 48 h or more, nanorods have a tendency to aggregate. Thus, purification is typically accomplished by pelleting via centrifugation, and the main pitfall is aggregation post-centrifugation. Since different sizes of particles will require different spin speeds to adequately drop out of solution, optimization for your particular nanorods is necessary. Centrifuging particles at too high of a speed or for too long can lead to the formation of aggregates, while under-centrifuging results in nanorod losses. This is especially true for nanorods with higher aspect ratios with absorbance outside of the visible spectra. Counterintuitively, higher aspect ratio rods are more stable in solution and, thus, require higher spin speeds for precipitation. Therefore, it is recommended that you check the UV/Vis spectra of supernatants before discarding. It is also important to be mindful of the final concentration of CTAB in your solution, as the removal of too much CTAB can cause the nanorods to become unstable. Typically, we recommend maintaining >10 mM surfactant through all purification steps. Particles can then be safely diluted to 1 mM CTAB for final storage. As seen in Figure 5, centrifugation can also be used for additional separation and purification of a sample with high shape impurities. While the majority of particles will remain together in a pellet (3), you can see on the fringes a differentiation in color (1 and 2) indicative of sphere and rod separation. While the method is inefficient, centrifugal separation can be utilized to increase purity and has been reported previously.61 Other groups have also explored multiphase centrifugal separations62 and flocculation63 techniques with some success.
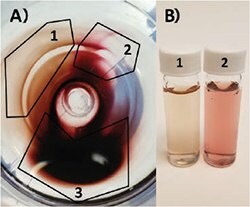
Figure 5.A) Top-down view of centrifuge tube containing gold nanoparticle pellet. Particles partially separate according to size and particle geometry: 1) Very high shape purity nanorod population. 2) Primarily nanosphere population. 3) Mix of both populations. B) Photo of (1) highly purified gold nanorods and (2) primarily spheres from pellet in A).
Incomplete Characterization (6)
In order to properly characterize nanorods, it is important to use multiple complimentary techniques to assess their quality. The most commonly used characterization tools are UV/Vis spectrophotometers, transmission electron microscopy (TEM), scanning electron microscopy (SEM), dynamic light scattering (DLS), and zeta potential. As discussed previously, UV/Vis spectroscopy is the most useful tool for measuring aspect ratio, shape purity, and rod quality for an ensemble of nanoparticles. However, UV/Vis provides little information on actual size. TEM and SEM are the most robust tools for verifying particle dimension, but without taking a proper number of images and doing so at multiple magnifications, it is easy to accidently bias the true average dimension of your particles. Figure 6 illustrates just how different the quality of a lot of nanorods can look based on what section of a TEM grid you choose to observe.47 Figure 6C demonstrates that particles can have a tendency to “self-sort” when drying on a grid. Groups of impurities such as spheres or cubes can separate from sections of rods leaving the false impression of a high shape purity synthesis.

Figure 6.TEM’s of gold nanorods illustrating selection bias. A) Section of TEM grid indicates high purity rod synthesis. B) Different section of same grid shown by (A) indicates a large number of impurities in sample. C) TEM demonstrates shape segregation drying pattern on TEM grid.
Techniques such as dynamic light scattering (DLS), which are typically employed for the characterization of spherical nanoparticles, rely on algorithms that are not designed for detection and sizing of nanorods due to their anisotropic geometry. However, measurement of the zeta potential can still act as a good marker of stability for nanorods stabilized by CTAB. Typically, stable as-synthesized gold nanorods after purification have a zeta potential >+30 mV; lower values indicate particle instability and a high likelihood of aggregation with time. Alternatively, gold nanorods can undergo ligand exchanges to be sterically stabilized by neutral moieties, such as polyethylene glycol (PEG). This method is often employed to remove CTAB and reduce particle toxicity, resulting in a zeta potential of ±5 mV.
Conclusions
The synthesis of gold nanorods via colloidal seed-mediated growth offers the user tunability over a wide range of aspect ratios and corresponding LSPR optical peaks, with the opportunity for easy volumetric synthesis scaling. However, this tunability comes at a price; a synthesis that requires precise control over a number of variables in multiple phases of the process. The extensive list of variables leads to difficulty in reproducibility and consistency of reactions, potentially frustrating researchers who are moving toward large-scale in vivo studies. Because of these challenges, small-scale manufacture can be costly to maintain.64 Therefore, many researchers opt to purchase nanorods rather than produce them in-house, as many companies now provide them as products with good quality control and standardized characterization.
References
Pour continuer à lire, veuillez vous connecter à votre compte ou en créer un.
Vous n'avez pas de compte ?