Barrier Formation and Permeability Assays using Millicell® Hanging Cell Culture Inserts
Read about
- Barrier Assay Overview
- What Do I Need to Run a Lucifer Yellow Assay?
- Selecting a Millicell® Hanging Cell Culture Insert
- TEER Detection Method
- TEER Application Notes, Guides, and Other Resources
- Lucifer Yellow Barrier Assay Sample Protocol
- Sample Barrier Assay Sample Data
- Barrier Assay Tips and Tricks
- Related Products
- References
Barrier Assay Overview
Cell culture barrier assays are used to model barrier tissues (kidney, lung, intestinal, etc.) in drug delivery, drug absorbance, wound healing, bacterial/viral infections, inflammation and other studies. These barriers can be formed in vitro when epithelial cells are seeded as a single cell suspension and grow together until fused into one tissue. Typically, this tissue will be one epithelial cell in thickness and is termed the epithelial monolayer. In standard barrier assays, cells are seeded onto porous membranes and observed noninvasively via trans-epithelial electrical resistance (TEER) measurements. TEER measurements are validated, label-free and fast techniques for indicating epithelial monolayer barrier formation before further evaluation and experimentation. Barrier formation is observed by the increase in resistance measure; a confluent epithelial monolayer will plateau at higher resistance values. For most barrier assays, an epithelial monolayer is the desired type of cell barrier because it models the single-cell thickness of lung-to-blood, kidney filtration, or intestinal absorption and can be used to analyze the paracellular space between epithelial cells. Once the integrity of the cellular barrier has been confirmed, these tissues can then be used in experiments that probe barrier permeability, disruption, or strength.
Tight junction pores are physiological gatekeepers of paracellular transport in epithelial tissues. Paracellular permeability assays assess monolayer barrier formation and tightness of paracellular tight junctions by measuring the amount of a molecule that passes through an epithelial cell monolayer in a given amount of time. This type of assay requires live cells and is usually non-destructive (e.g., Lucifer yellow paracellular permeability assay). Immunostaining and tissue immunocytochemistry (ICC) are commonly used for visualization, pre-empting the need for electron microscopy. Many of these analyses are focused on barrier pass-through tracked as dye, drug conjugates, or amount of target protein transferred. Alternative analyses involve visualizing the membrane using stains such as crystal violet, fluorescent antibody staining, and ICC.
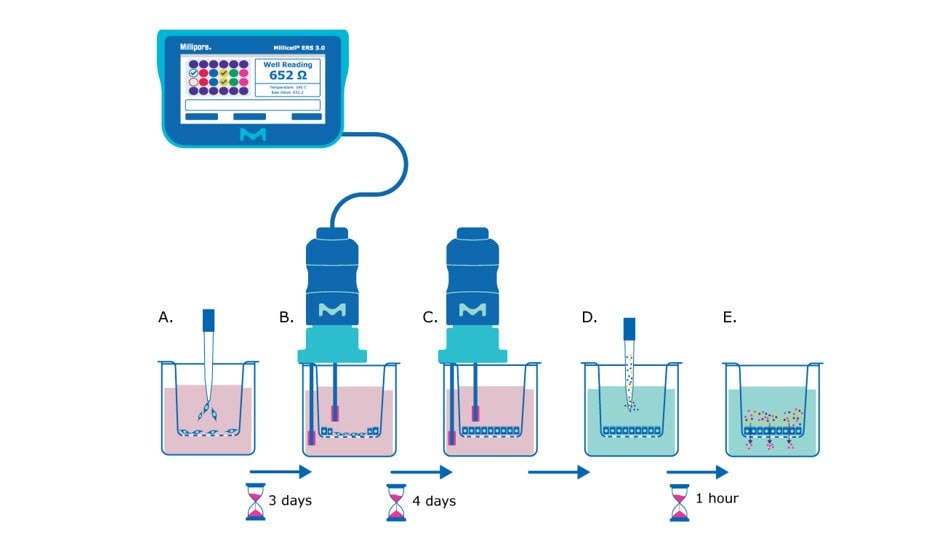
Figure 1. Barrier assay principle using Millicell® hanging cell culture inserts. A) On day 0, epithelial cells are seeded in single-cell suspension onto the Millicell® hanging cell culture insert membrane. B) After several days (~3 days after seeding for MDCK cells) the cells will be confluent enough to begin taking TEER measurements, assessing the epithelial cell monolayer formation based on the resistance value. C) TEER measurements can be taken daily or every other day to track monolayer barrier formation until cells are seen to plateau at a consistent resistance value (~7 days after seeding for MDCK cells). D) At this point the media is exchanged for barrier assay liquid (Hank’s balanced salt solution) and permeability assay dye/molecule (e.g., Lucifer yellow dye) is added to the apical side of the membrane. E) The small molecules are incubated with the epithelial monolayer at 37°C for recommended protocol length (e.g., 1 hour), and then the liquid from the basolateral side of the membrane insert is evaluated for the amount of pass-through of the chosen molecule.
What do I need to run a Lucifer yellow barrier assay?
- Millicell® hanging cell culture insert with 0.4 or 1 µm pore size (see Selecting a Millicell® Hanging Cell Culture Insert below)
- Receiver plate (CLS353046, CLS353043, CLS353047)
- Lucifer yellow dye
- Hank’s balanced salt solution
- Millicell® ERS 3.0 Digital Voltohmmeter
- Microplate reader
- 96-Well black/clear bottom microplate
Selecting a Millicell® hanging cell culture insert
Barrier assays are performed on hanging inserts as porous membranes offer vastly improved characteristics over standard polystyrene surfaces for what is called 2.5D cell culturing. Membranes in these inserts differ in terms of pore size, which becomes important for different assay types. For example, migration assays will have the widest range of pore sizes based on the cell type involved in the experiment.
TEER Detection Method
The Millicell® ERS 3.0 voltohmmeter uses low current and voltages to allow for non-destructive testing for epithelial monolayer confluence in cell cultures. TEER instruments produce a low AC current that avoids electrode metal deposits and adverse effects on tissues which can otherwise be caused by higher DC currents. Confluence of a cellular monolayer is determined by an increase or a plateau in tissue resistance detected using TEER readers.
MDCK culture media
- Dulbecco’s MEM with high glucose
- 10% FBS
- 1% (1x) NEAA
- 1% Penicillin/streptomycin
- 4 mM L-glutamine
Seeding cells onto a 24-well Millicell® hanging cell culture insert
- Culture MDCK cells until they are 60-80% confluent.
- Aspirate media from the culture flask.
- Add sterile PBS for 3 minutes at room temperature.
- Aspirate liquid from the culture flask.
- Add trypsin to the culture flask and incubate in a 37°C, 5% CO2 cell culture incubator.
- Stop the trypsin reaction by adding equal parts complete cell culture media to the flask.
- Collect liquid suspension and spin down in the centrifuge at 300 x g for 3 minutes.
- Resuspend cells in 1 mL of cell complete culture media (see MDCK culture media recipe above).
- Count cells using trypan blue and hemocytometer. Mix 90% trypan blue with 10% cell suspension (e.g., 180 µL trypan blue and 20 µL cell suspension).
- Count live cells on the hemocytometer and calculate cells per milliliter.
- Add 900 µL of complete media to the basolateral side of the 24-well membranes.
- Resuspend cell suspension in appropriate volumes to dispense 200 µL of suspension containing the desired cell seeding density (e.g., 1,250 cells/insert, 2,500 cells/insert, 5,000 cells/insert).
- Place in the 37°C, 5% carbon dioxide cell culture incubator and change media every other day.
NOTE: Best practice is to pre-warm complete cell culture media before adding to Millicell® hanging cell culture insert and receiver plate.
Measuring cell resistance
- Allow plate reach to reach room temperature (15-30 minutes).
- Load plate map and assign wells to groups on the Millicell® ERS 3.0 Digital Voltohmmeter (see p. 11 of user guide).
- Test the Millicell® ERS 3.0 Digital Voltohmmeter using the verification adapter (see p. 22 of user guide).
- Set the MODE switch to Ohms and turn the Power switch On.
- Immerse the electrode so that the shorter tip is in the apical side of the Millicell® hanging cell culture insert, and the longer tip is in the outer well. The shorter tip should not contact cells growing on the membrane and the longer tip should just touch the bottom of the outer well. To ensure stable and reproducible results, make sure that the electrode is held steady at a 90° angle to the plate and insert bottom.
NOTE: If rinsing is required between measurements to prevent sample carryover, use cell culture media rather than distilled water. - Record the resistance.
- Determine blank resistance by adding media or electrolyte solution to a blank well/cell culture insert (i.e., the cell culture insert without cells and complete cell culture media).
- Measure the resistance across the blank insert and use this value as a “zero” or “background” resistance level. To obtain true tissue resistance, subtract the resistance reading across the blank insert from the resistance reading across the tissue (cell culture insert with cells).
- The resistance is inversely proportional to the area of the tissue. The larger the membrane, the lower the resistance.
Unit Area Resistance (Ω × cm2) = Resistance (Ω) × Effective membrane Area (cm2)
NOTE: Resistance can vary based on sample temperature, pH, and electrode depth in solution.
TEER Application Notes, Guides, and Other Resources
- Using Immortalized 16HBE14o- Human Bronchial Epithelial Cell Lines to Model Respiratory Lung Diseases
- Millicell® Cell Culture Inserts & Plates
- The better way to TEER
- MultiScreen® Caco-2 Assay System
Lucifer Yellow Barrier Assay Sample Protocol
Steps
- Aspirate media from the apical and basolateral sides of the membrane/well.
TIP: Be careful not to touch or poke the membrane while aspirating. - Wash/rinse membrane and well with prewarmed Hank’s Balanced Salt Solution (HBSS).
TIP: Prewarmed HBSS has the least impact on the cell monolayer; the use of PBS will be more harmful and cause a greater percentage of dye passthrough. - Aspirate HBSS from the apical and basolateral sides of the membrane/well.
TIP: Be careful not to touch or poke the membrane while aspirating. - Dispense 900 µL HBSS to the basolateral side of the membrane.
- Dispense 200 µL of Lucifer yellow dye (100 µg/mL) to the apical side of the membrane.
TIP: Be very careful not to accidentally add Lucifer yellow dye or spill/drip into the basolateral compartment. This will disrupt assay result accuracy. Do not poke the membrane when pipetting. - Place in 37°C, 5% CO2 incubator for 1 hour. Increase incubation time to 2-3 hours for low porosity membranes like the 0.4 µm clear Millicell® inserts (PCHT24H48).
TIP: For best results keep plate flat and do not shake. - Use this time to make standards for the assay plate, which identify your sample values.
a. Make a 100% standard [Std(100)] by combining:
200 µL of Lucifer yellow Dye (100 µg/mL) with 900 µL of HBSS.
b. Dilute standards 1:1 in a serial dilution.
500 µL of Std(100) with 500 µL of HBSS would make the Std(50)
c. Lowest standard (besides 100% HBSS) should contain 0.39% Lucifer yellow (this would make nine standards and a tenth for HBSS
"zero").
d. Add 100 µL of each standard to a fluorescence spectroscopy plate (in triplicate). - Remove plate from incubator and collect 100 µL of fluid from the basolateral side of the membranes to place into the fluorescence spectroscopy plate.
TIP: For best results, do this in triplicate so that results can be averaged as technical replicates. - Read fluorescence spectroscopy plate on a plate reader and determine the fluorescent absorbance using 485 nm excitation and 535 nm emission wavelengths.
- Use the generated standard curve to calculate the Lucifer yellow concentration in each well. These values can then be used to determine the percent pass-through for each sample.
RFU = relative fluorescence units assigned by the plate reader.


Figure 3. All membranes depicted are high-density (1×108) 0.4 µm pore size PET 24-well membranes. These results compare Millicell® hanging cell culture inserts (PTHT24H48) to two comparable brands of inserts. MDCK cells were seeded at the same seeding density in parallel across all three manufacturers. The three brands of inserts are not significantly different from each other on day 7 after seeding and follow a similar kinetic for the formation of a monolayer.

Figure 4. Lucifer yellow permeability assays test the formation of the cellular monolayer and tight junctions between cells. All membranes depicted are high-density (1 x 108 pores) 0.4 µm pore size PET membranes in 24-well formats. These results compare the Millicell® hanging cell culture insert (PTHT24H48) to two other brands of inserts. This assay was performed on Day 7 of the assay in Figure 3. Barrier formation is deemed successful if there is less than 5% pass-through of the dye from the apical side of the membrane into the basolateral side of the membrane (pass-through depicted in the final panel in Figure 1). There were no statistical differences between these inserts.

Figure 5. TEER values are dependent on starting seeding densities, which correlate with the confluence of cells on the Millicell® hanging cell culture insert. Higher cell seeding densities will form a cellular monolayer faster. Depending on the experiment, it might be more beneficial to have higher or lower seeding densities depending on the study’s focus. In the example above, MDCK cells were seeded from the same stock and were measured for resistance on the days indicated. All measurements were taken on the Millicell® ERS 3.0 digital voltohmmeter with Millicell® hanging cell culture inserts (PTHT24H48).
Barrier Assay Tips and Tricks
- Choose a membrane that best fits your cell type and assay (see Table 1 above)
- Change media every other day to ensure cells have enough nutrients and maintain vital cell signaling for monolayer/tight junction formation.
- Do not leave cells outside of the incubator for prolonged periods of time before assays. This can negatively impact Lucifer yellow permeability assay results as well as other similar assays.
- Make sure the electrodes on the TEER probe are fully submerged in media for the most stable readings.
- Hold the TEER probe at the same depth in each well/insert for consistent and comparable readings.
- To ensure stable and reproducible results, make sure that the electrode is positioned at a 90° angle to the plate insert.
- Resistance can vary with temperature. TEER readings increase (higher ohms Ω) at lower temperatures (lower °C). Be sure to wait for samples to acclimate to room temperature before taking readings.
- Resistance is affected by media pH. Higher carbon dioxide content will be present in samples that are pulled immediately out of an incubator and is associated with lower resistance values. The amount of carbon dioxide will equilibrate with the atmospheric carbon dioxide while acclimating to room temperature.
- Clean TEER electrodes periodically with an enzymatic detergent such as Tergazyme® to eliminate protein buildup on the electrode from cell culture media. This cleaning procedure can be found in the Millicell® ERS 3.0 user guide.
- The Millicell® ERS 3.0 model has a cable that is more vulnerable to interference from radio waves. These come from cell phones, laptops, computers, radiation equipment (MRI, x-ray, etc.), and even smart watches. Consider moving testing area to an area with the least amount of these disturbances.
- Never put the Millicell® ERS 3.0 voltohmmeter or its electrode probe under UV light. This will damage the electrode sensor and lead to inaccurate, variable, or failure to take readings.
- If rinsing is required between measurements to prevent sample carryover, use cell culture media rather than distilled water.
- Be careful not to touch or poke the membrane while aspirating.
- During a Lucifer yellow permeability assay, prewarmed HBSS has the least impact on the cell monolayer; the use of PBS will be more harmful and cause a greater percentage of dye pass-through.
- Be very careful not to accidentally add Lucifer yellow dye or spill/drip into the basolateral compartment. This will disrupt assay result accuracy. Do not poke the membrane when pipetting.
- Once Lucifer yellow dye is added to the membrane, for best results keep plate flat and do not shake.
- At the end of the Lucifer yellow permeability assay, collect basolateral liquid after mixing (pipette up and down), then run passthrough results in technical triplicates for the best outcome.
1 - 20
21 - 40
41 - 60
61 - 80
81 - 82
Para seguir leyendo, inicie sesión o cree una cuenta.
¿No tiene una cuenta?