Selectivity Study of Stationary Phases for Hydrophilic Interaction Chromatography (HILIC) and Multivariate Analysis for Material Classification
C. Corman - Senior Research Scientist, A. Kumar - Senior Research Scientist
Abstract
Fifteen Hydrophilic Interaction Chromatography (HILIC) column phases were evaluated for their chromatographic properties. Nine specific factors representing retention, hydrophilicity, hydrophobicity, shape selectivity, and ion exchange capabilities were investigated to characterize the phases. Their properties are visualized by spider web graphs and a phase clustering is done by multivariate analysis, and principal component analysis. This is a helpful tool for selecting phase chemistries for HILIC method development.
Section Overview
INTRODUCTION
The need for chromatographic materials to retain and separate polar and hydrophilic molecules is a continuously growing trend. A popular separation technique, Hydrophilic Interaction Chromatography, or HILIC as it is commonly abbreviated, is an excellent choice for the separation of these types of analytes. In this separation mode, analytes retain and elute based on a multitude of factors –partitioning into and from adsorbed water layer(s) of the hydrophilic stationary phase surface, ionic interactions with charged analytes, hydrogen bonding, dipole-dipole interactions, and adsorption in some cases. Due to the multifunctional nature of HILIC’s retention mechanisms, the choice of HILIC column stationary phase is very important.
Herein, we present a column classifying approach developed by Ikegami et al1 which gives information about retention and selectivity properties (summarized in Table 1) for HILIC phase chemistries. In addition, the multivariate analysis allowed for the clustering of stationary phases based on their chemical modification.
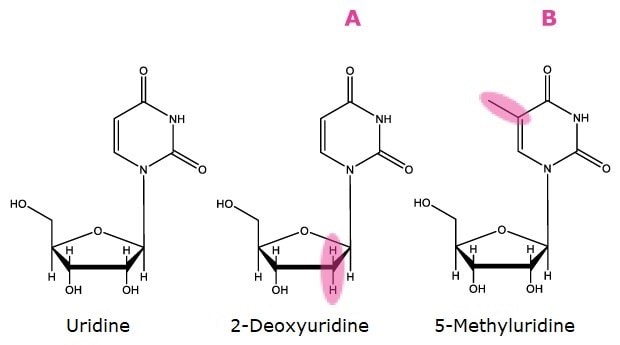
Markers of Hydrophobicity/Hydrophilicity. A). Deoxygenated at 2 position, B). Addition of methyl group
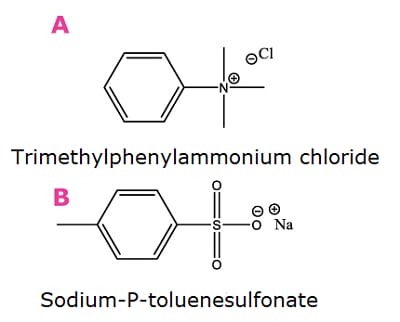
Markers of ion exchange. A). Cationic probe, B). Anionic probe
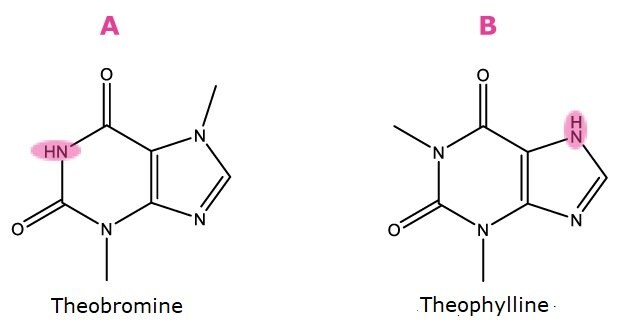
Markers of acidity/basicity. A). Proton location, pKa ∼ 10, b). Proton location, pKa ∼ 8.6
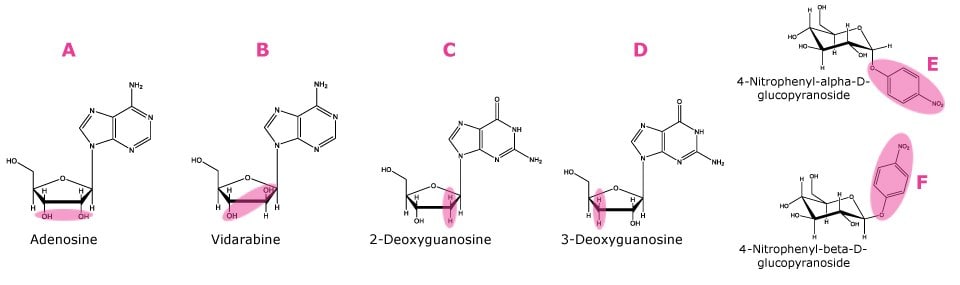
Markers of shape selectivity. A). cis hydroxyl groups, B). trans hydroxyl groups, C). Deoxygenated at 2 and hydroxyl group at 3 position, D). Deoxygenated at 3 and hydroxyl group at 2 position, E) Axial nitrophenyl group, F). Equatorial nitrophenyl group
Figure 1. Compounds used as markers for HILIC factors as described in Table 1.
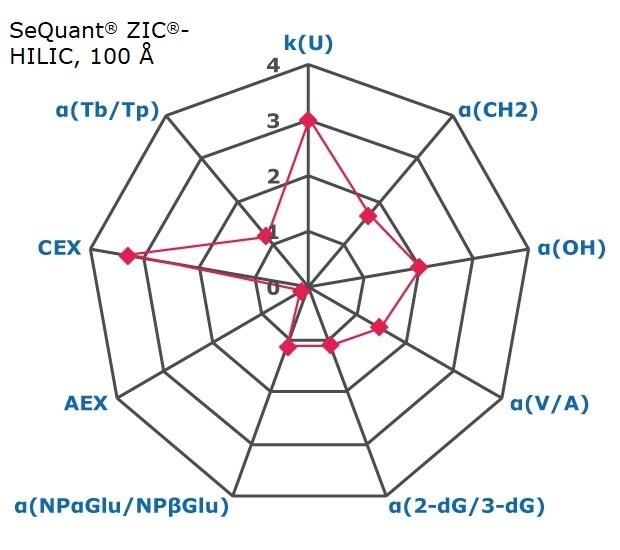
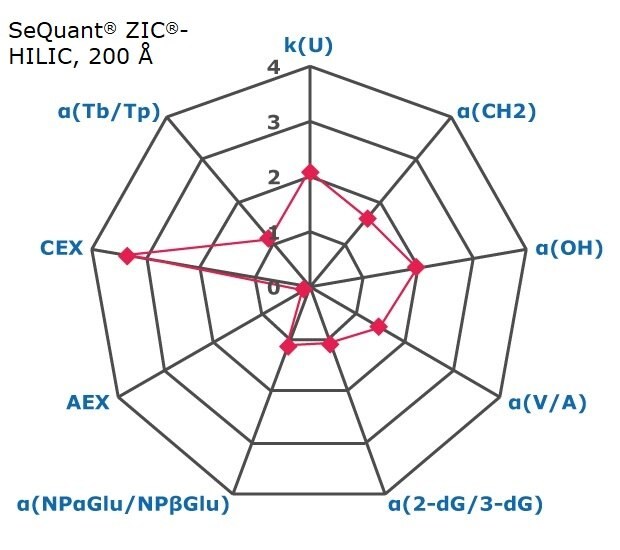
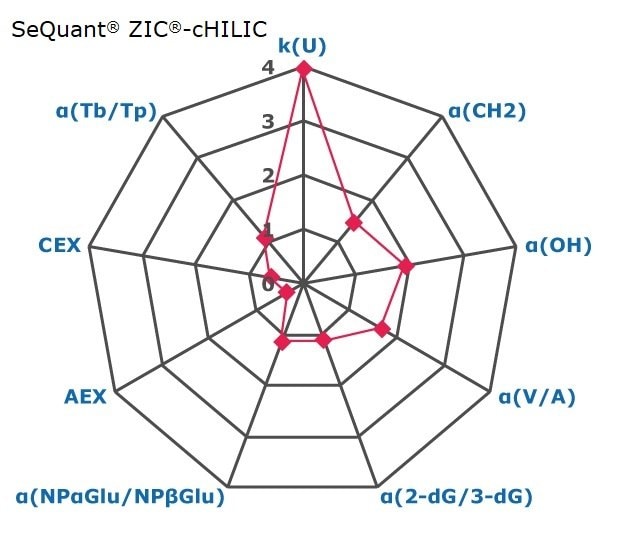
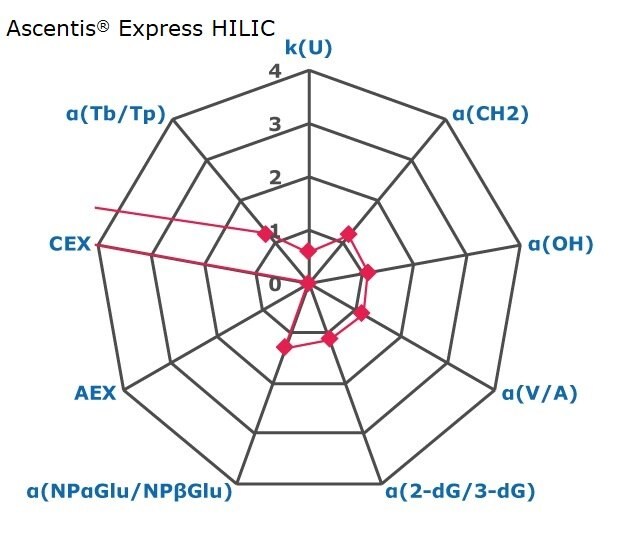
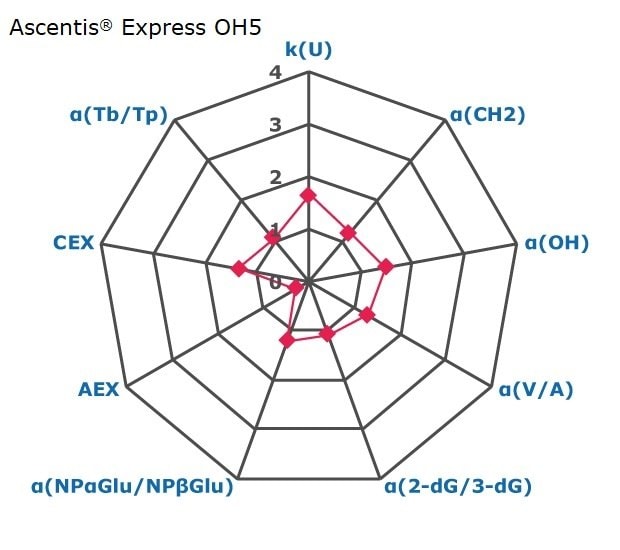
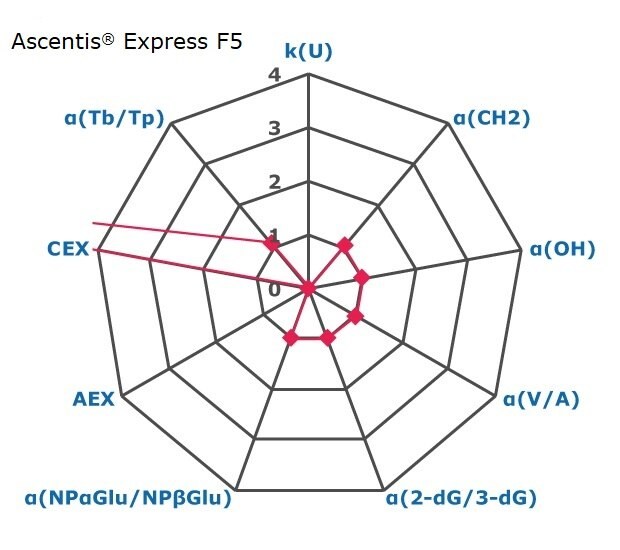
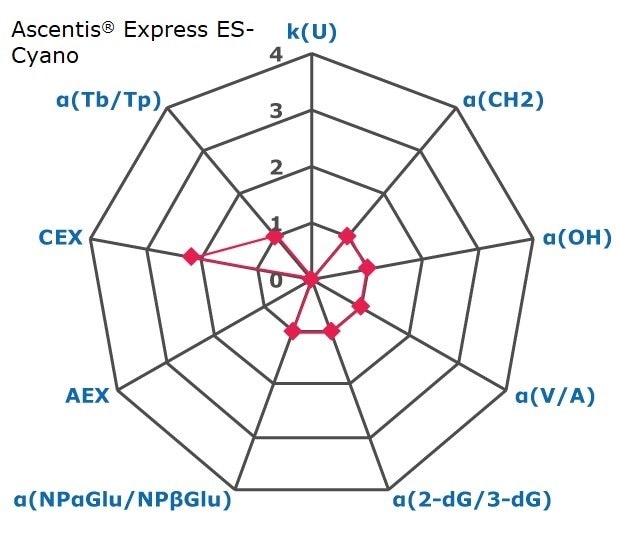
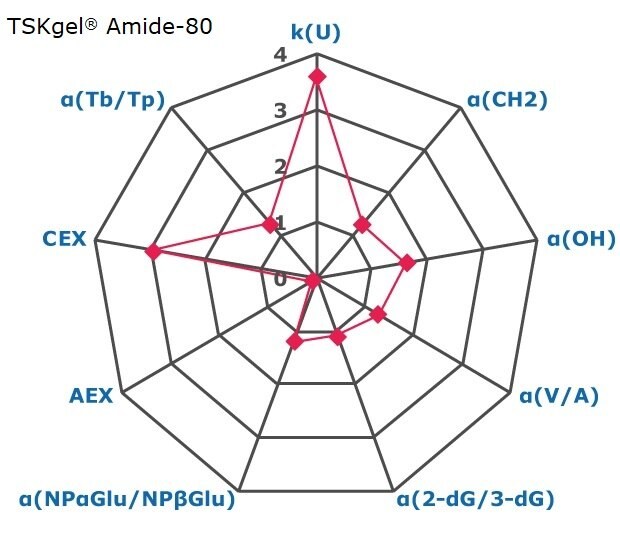
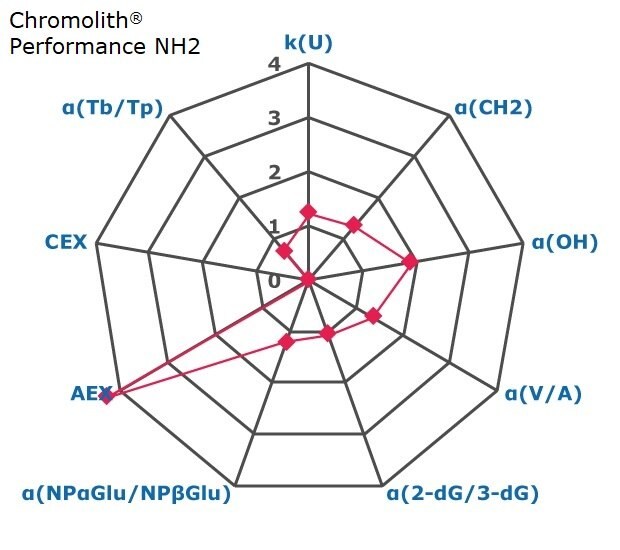
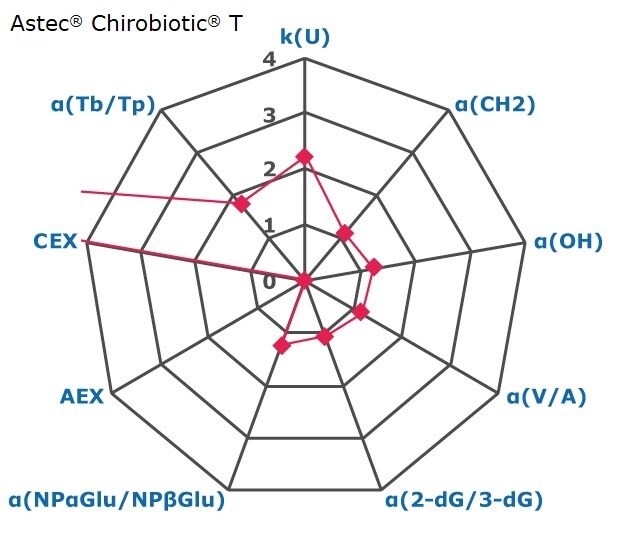
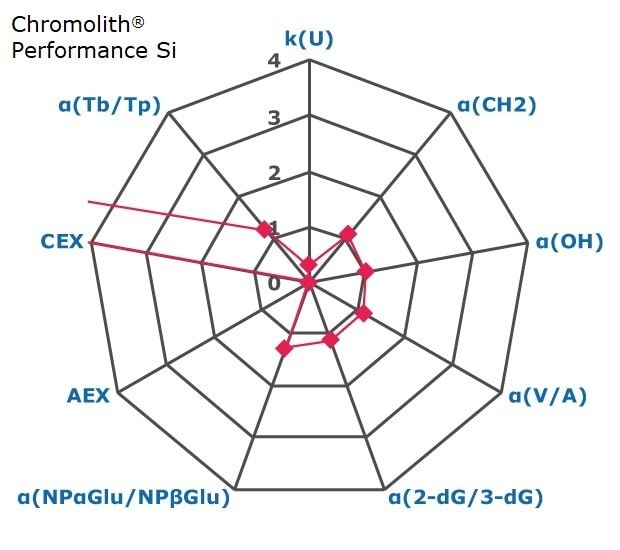
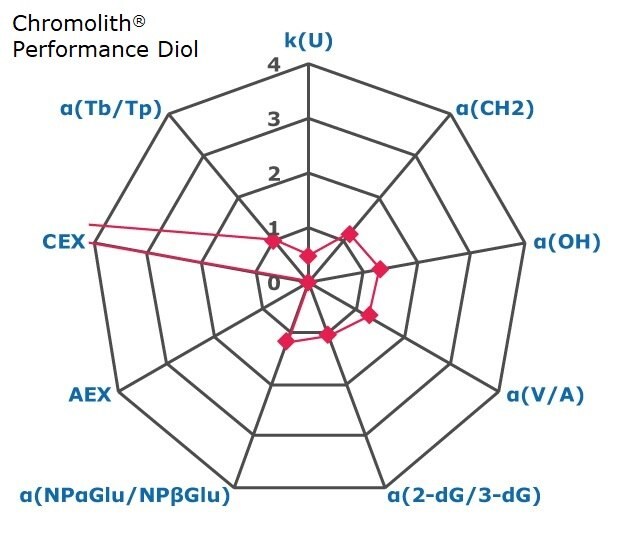
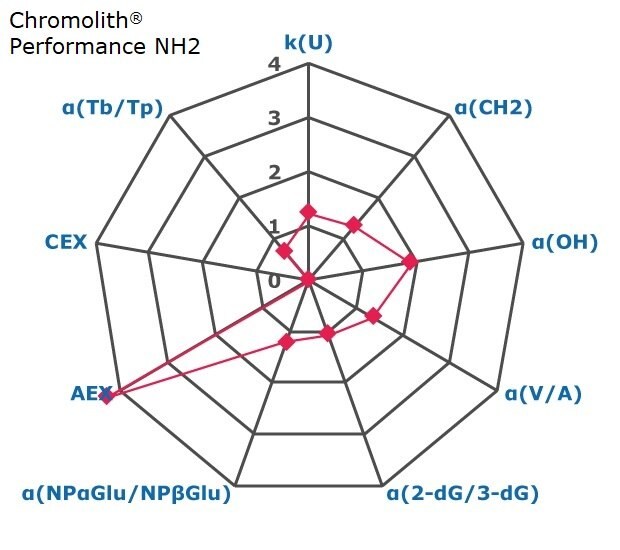
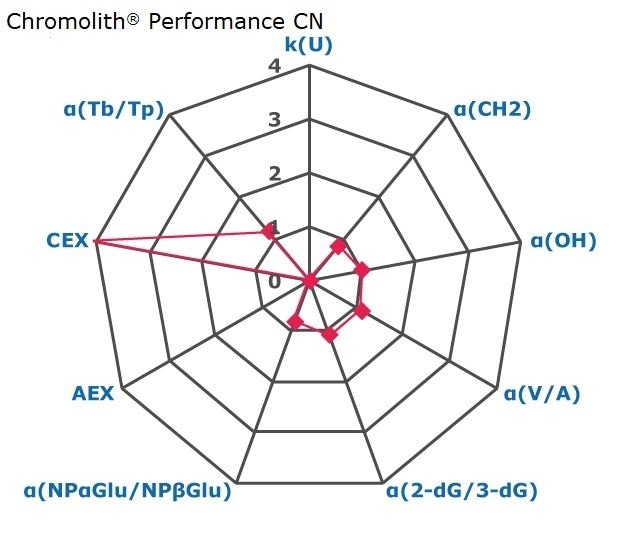
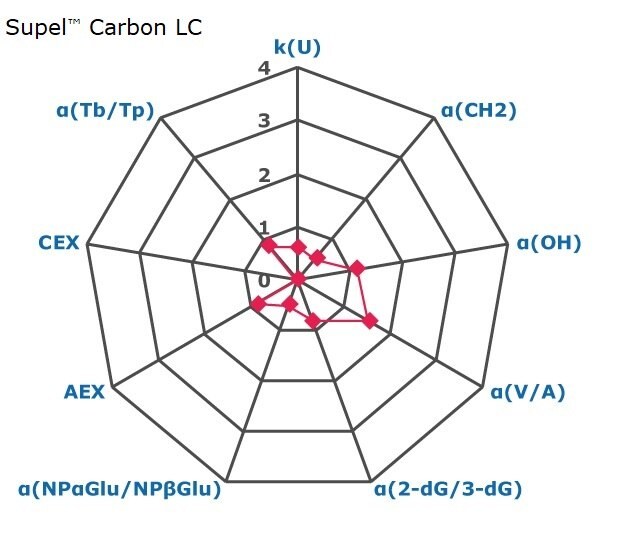
Figure 2. Results on HILIC factors for 15 stationary phases.
Some general findings from the evaluated stationary phases could be broken up into two main categories:
- Phases with weak ion-exchange mechanisms, but strong retention of neutral hydrophilic probes – Zwitterionic and Amide phases.
- Phases with predominantly ion-exchange mechanisms and weak or no retention towards the neutral hydrophilic probes - Bare silica, pentafluorophenyl, cyano, diol, and amine phases.
In Figure 3 the phases are graphically positioned relatively to each other for their strength in hydrophilic and ion exchange retentivity. For Hydrophilic Retention the cyano, fluoro, and carbon phases show the least retention while the zwitter ionic and amide phases the most. It should be noted that the Carbon LC (PGC) is typically operated under RP conditions to retain highly polar compounds. The here for comparability chosen unified HILIC mobile phase conditions are not the optimal for operating this phase type. The PGC column was also the shortest (5 cm) in this comparison. Hence results under RP conditions and a longer column will differ.
Regarding ion-exchange retention the amine phases showed strongest anion exchange followed by the carbon phase, while bare silica, fluoro and tecoplanin, diol, cyano phases stronger cation exchange. Zwitter ionic are more balanced towards in the middle for ionic retention with slight trend to cation exchange.
Figure 3. Comparison of hydrophilic and ion exchange retention properties of investigated HILIC phases.
In terms of overall selectivity, the zwitterionic stationary phases are the most comprehensive. For this reason, the use of zwitterionic columns is highly recommended as a good starting point for method development. However, if mainly ionizable analytes will be present, use columns with strong ion-exchange mechanisms. Typically, when ion exchange is present it tends to be the dominant retention mechanism. Bare silica columns and pentafluorophenyl phases are a sensible choice for cation exchange while amine phases are well suited for anion exchange. Curiously, the porous graphitic carbon phase also demonstrates anion exchange properties.
The use of multivariate analysis, principal component analysis (PCA), was performed using Minitab software for further characterization of the stationary phases (Figure 4). This resulted in the clustering of the data set which could then be grouped by their chemical modification. Although the use of PCA may not be necessary for general method development purposes, it has been extremely useful in the classification of new HILIC materials.

Figure 4.PCA score plot of the 15 evaluated HILIC phases.
CONCLUSION
Fifteen stationary phase materials were characterized for HILIC. The testing methodology shown has been useful for the initial screening of HILIC materials. By and large, the sorbents could be classified as phases that demonstrate strong hydrophilic retention or phases with weak retention properties but strong ion exchange retention. With this in mind, it is recommended to have a set of three to four columns when screening for HILIC method development – zwitterionic, bare silica, amine, and amide phases. Details about analyte properties (logD, pKa, charge state, etc.) will give further intuition as to what column chemistry is reasonable to start with first. Furthermore, characterization with multivariate tools was shown as an effective approach to classify materials based on their chemical modification.
References
Para seguir leyendo, inicie sesión o cree una cuenta.
¿No tiene una cuenta?