Use of Stabilizers and Surfactants to Prevent Protein Aggregation

Read more about
- Critical Factors in Long-Term Stability of Proteins
- Manufacturing Conditions and Drug Product Handling
- Stabilizers to Protect Against Mechanical Stress: Surfactants and Cyclodextrin
- Stabilizers Against Thermal Stress: Sugars, Sugar Alcohols and Amino Acids
- How Purity and Quality of Raw Materials can De-Risk Drug Development
- The Need for an Expanded Excipient Portfolio
Biomolecules, such as proteins or mAbs, are inherently delicate structures stabilized by a large number of individually weak forces. The necessary long shelf life and various stresses typically occurring during manufacturing and handling need to be managed by using excipients to create a stable, and thus safe, drug product. Said excipients not only have their specific function but may also have unique quality attributes that positively affect protein stability.
Critical factors in long-term stability of proteins
Monoclonal antibodies (mAbs) and plasma-derived protein products are used to treat a variety of acute and chronic illnesses. For a typical drug product, a shelf life of two years can be enabled by its formulation, but given the very nature of proteins, this can be a challenging task. The thermodynamic fluctuations a protein will undergo during its shelf life may lead to a population of misfolded proteins which can lead to the formation of aggregation nuclei and loss of function, both of which affect therapeutic efficacy. Aggregation can also lead to safety issues for patients due to their potential to trigger an immune response. With use of an appropriate buffering substance and the addition of stabilizing excipients, the frequency of these thermodynamic fluctuations can be reduced, and long-term stability of the protein can be improved.
In addition to the thermodynamic fluctuations to which proteins are exposed, storage of antibodies in a glass vial can, over time, lead to aggregation as a result of surface effects such as adsorption. Proteins may show an amphiphilic character with hydrophobic amino acid residues more likely to be located in the protein’s core, while hydrophilic amino acid residues on the surface facilitate protein solubility. The interaction of proteins with hydrophobic interfaces including liquid-solid (e.g., the glass wall of a vial), liquid-air or liquid-ice leads to adsorption processes. As a result, a highly concentrated protein film can result in which the proteins may also undergo partial unfolding. The rupture of these protein films due to mechanical impulses such as shaking of the vial, can lead to the formation of large protein aggregates.
Manufacturing conditions and drug product handling
In addition to challenges that arise from the nature of proteins and long-term storage in glass vials, manufacturing conditions and drug product handling can impact stability.
- pH is a critical factor in drug formulation and its effect on protein stability must be considered.
- Pumping the feedstream through tubing during purification or fill and finish can cause mechanical stress on the drug substance.
- Shaking of vials during transport and storage of the drug at room temperature e.g. prior to administration can affect stability.
Use of proper excipients can help guarantee long-term stability of the protein drug substance, ensure manufacturability, and support safe handling.
Stabilizers to protect against mechanical stress: surfactants and cyclodextrin
To prevent surface or mechanically induced aggregation because of pumping or shaking, surfactants or cyclodextrin can be used.
As described above, surface-induced protein aggregation is triggered by protein adsorption to hydrophobic interfaces such as liquid-air and liquid-ice interfaces. The highly concentrated protein film that is formed due to the adsorption processes can lead to protein particle formation upon a subsequent mechanical stress such as agitation. Surfactants can prevent this type of aggregation via two distinct mechanisms (Figure 1).
Surfactants are surface active substances and compete with proteins for the adsorption at these interfaces. Surfactants either occupy the space on the surface and prevent protein adsorption or replace adsorbed proteins, returning them to the liquid phase, inhibiting the protein film formation and reducing the possibility of aggregation (Figure 1B).
Surfactants can also improve protein solubility by directly interacting with the target protein, acting as chaperones (Figure 1C). Some antibodies may expose hydrophobic patches to the aqueous solvent; surfactants can bind to the antibody in these structural regions shielding them from the hydrophilic environment. By covering these hydrophobic patches on the surface of the protein, surfactants prevent antibody adsorption to a surface or formation of aggregation nuclei with other antibodies.
A: Protein Adsorption to Interfaces
B: Competitive Surface Adsorption
C: Surfactant – Protein Binding


Figure 1. Protein adsorption to interfaces which triggers the formation of a protein film and subsequent aggregation (A) is prevented by adding surfactants through two mechanisms: By competitive surface adsorption with the surfactant molecules covering hydrophobic interfaces (B) or direct binding of the surfactants to the protein molecules, solubilizing them in the liquid phase (C).
Polysorbate 20 and 80 are commonly used surfactants and are present in 90% of approved monoclonal antibody formulations.1 Depending on the excipient that is used and the protein to be formulated, concentrations of 0.01% and 0.1% are used. Poloxamer 188 is a different type of surfactant, but is used in the same concentration regime.
While cyclodextrins cannot be classified as surfactants, these molecules have been shown to stabilize proteins against surface- and agitation-induced stress at concentrations as low as 0.35% (2-Hydroxypropyl)-β-cyclodextrin is a cyclic oligosaccharide composed of seven α-glucopyranose monomers, forming a hollow truncated cone with a hydrophilic surface and a hydrophobic cavity. These molecules show some surface activity, which may be involved in protein stabilization to some extent, even though their surface tension-reducing capabilities are limited.
In a forced degradation study various proteins were exposed to stirring, shaking, peristaltic pumping and freeze/thaw stress. Figure 2 shows that high-purity polysorbate 20 and polysorbate 80, poloxamer 188, and cyclodextrin prevented protein aggregation resulting from all stress types that were applied to trigger surface- or agitation-induced protein aggregation. Successful stabilization was demonstrated by 100% monomer recovery and low turbidity. For surfactants, this was achieved using typical concentrations; for cyclodextrin, a concentration of 0.35%, was shown to be efficient.
mAbE under Shear Stress
mAbE under Shaking Stress
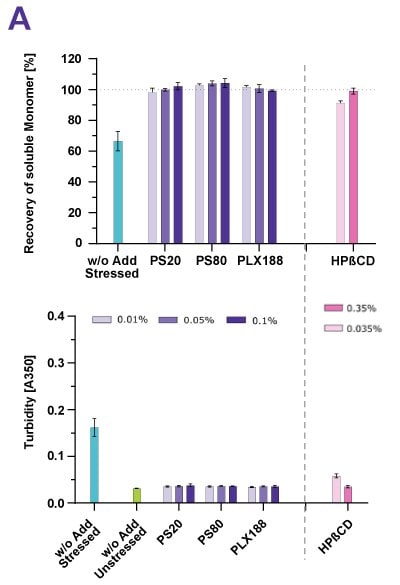

Figure 2. Cyclodextrin (HPβCD) effectively stabilized the mAb whether the protein was under shear stress (A) or shaking stress (B).
Stabilizers against thermal stress: sugars, sugar alcohols and amino acids
To stabilize proteins against unfolding due to factors such as thermal stress or a pH shift, sugars, sugar alcohols, and amino acids can be used. These molecules shift the equilibrium between native and partially unfolded proteins in a way that increases stability.
There are two mechanisms by which the respective chemical can function (Figure 3A):
- An excipient that is preferentially excluded from the protein surface strengthens the hydration shell around the antibody, shifting the equilibrium towards the natively folded state (Figure 3B).
- If a chemical is preferentially binding a partially unfolded protein, it prevents aggregation and further degradation (Figure 3B). By doing so, the excipients enable subsequent refolding to the native state over time or upon removal of the stress condition.
A: Without Excipient
B: Preferential Exclusion
C: Preferential Binding
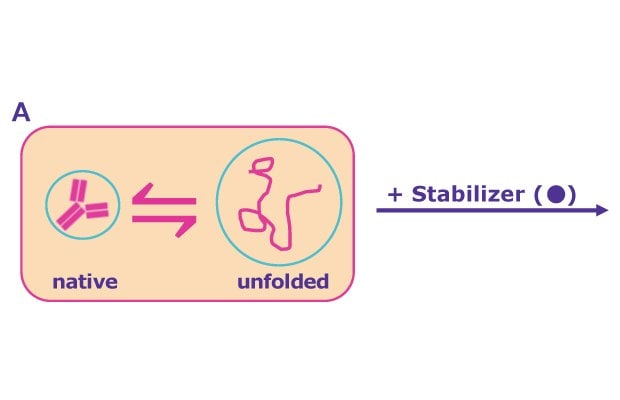

Figure 3. In solution, a dynamic equilibrium between the natively folded and (partially) unfolded protein state exists - the latter of which is susceptible to aggregation (A). Stabilizers can shift the equilibrium towards the natively folded state through preferential exclusion of the excipient from protein surface and hydration of the protein molecule (B). Other stabilizers act through preferential binding to the partially unfolded state of the protein, preventing aggregation and further degradation and later refolding (C).
Several amino acids can be used to stabilize proteins. The glycine backbone, which is common in all amino acids, has well-known stabilizing properties. Arginine includes a guanidinium group, which has a delocalized positive charge and as such, interactions with aromatic residues can help solubilize the target protein or reduce the viscosity of a formulation. Histidine is another stabilizing amino acid and is commonly used as a buffer for monoclonal antibody formulations.
Sugars and sugar alcohols are used as protein stabilizers against thermal and thermodynamic effects including sucrose, trehalose, and myo-inositol. To demonstrate the stabilizing impact of sugars, a variety of antibody drug products, including a fusion protein and two ADC-mimics, were exposed to forced degradation conditions. To ensure comparability of results, a temperature of 10 °C below the individual thermal transition midpoint of each protein was chosen. Samples were examined for turbidity, monomer content, monomer purity, and aggregates as well as by visual inspection. Figures 4A-D show an example data set for the antibody Denosumab.
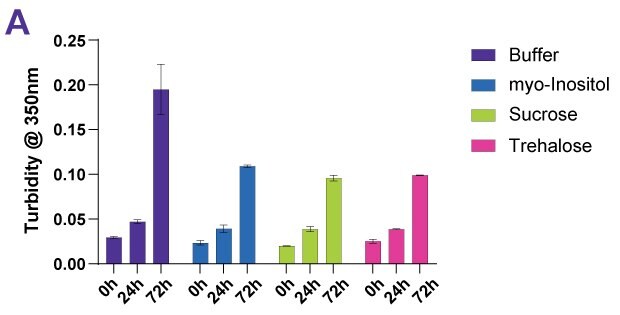
Figure 4A.Effect of different protein stabilizers following forced thermal degradation of Denosumab as measured by turbidity.

Figure 4B.Effect of different protein stabilizers following forced thermal degradation of Denosumab as measured by monomer content.
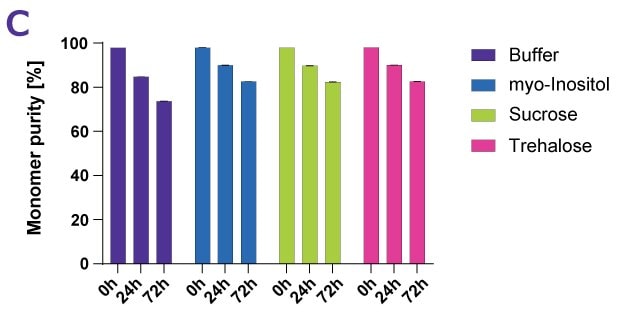
Figure 4C.Effect of different protein stabilizers following forced thermal degradation of Denosumab as measured by monomer purity.

Figure 4D.Effect of different protein stabilizers following forced thermal degradation of Denosumab as measured by percentage of aggregates.
Table 1 shows the comparable protein stabilization effect of the sugar alcohol myo-inositol and sucrose and trehalose.
In addition to their applications in classical liquid formulations, sucrose and trehalose are also used as stabilizers during lyophilization. Together with bulking agents such as sorbitol or mannitol, the sugars form a glass matrix which stabilizes proteins by water replacement and vitrification. During the drying process, stabilizers can replace water in hydrogen bonding with a protein; as such, the native structure can be maintained even in the absence of water. Vitrification stabilizes molecules by forming a rigid glass-matrix. In this matrix, thermodynamic equilibrium dynamics are no longer relevant, and proteins are stabilized kinetically. While sugars such as sucrose and trehalose provide a reasonably high glass transition temperature, other excipients can be used to strengthen hydrogen bonding to the immobilized protein.
Sorbitol and mannitol are examples of commonly used bulking agents. Besides acting as protein stabilizers, these bulking agents can reduce drying time, making the lyophilization process more efficient. They may also act as reconstitution enhancers, depending on the way the formulation has been lyophilized. It should be noted, however, that bulking agents are typically plasticizers which reduce the glass transition temperature of the formulation. For a lyophilized formulation to be stable, it must be stored at a temperature of approximately 30 °C below the glass transition temperature. During formulation development, the freeze-drying process, as well as the choice and the amount of excipients that are used, must be optimized to create a stable formulation.
The purity and quality of raw materials can de-risk drug development
The final drug product must meet high safety standards and be compliant with numerous regulations. For example, there are strict limits on bioburden and endotoxin levels in a drug product. High-quality raw materials produced under controlled conditions can further minimize the risk of failing to meet safety requirements. In addition to bioburden and endotoxin levels, additional excipient-specific quality attributes are equally important.
Polysorbates, for example, are prone to oxidation and hydrolysis; the resulting degradation products include fatty acids and peroxides which negatively impact protein stability. There is a trend in the industry to develop more stable surfactants and ultimately replace polysorbates. While these novel solutions are still under development, it is recommended to use polysorbates in their highest purity grade, if poloxamer 188 or cyclodextrin cannot be considered.
Additional quality attributes of sucrose are also critical. Some sucrose batches may contain nanoparticulate impurities that can be mistaken for protein aggregates and cause false analytical results. Additionally, these nanoparticular impurities can form nuclei for protein aggregation. Producing sucrose in a controlled environment can reduce the amount of nanoparticulate impurities and minimize the risk of genuine or analytically false protein aggregation.
When used as a powder, glycine has a high tendency for caking; the resulting glycine cakes can be difficult to break apart, making their use manufacturing quite cumbersome. Granulated glycine has a greatly reduced tendency to form cakes and offers better flowability and quick dissolution behavior making it more amenable for use in bioprocessing.
The need for an expanded excipient portfolio
During manufacturing and final use, a drug product is exposed to numerous factors that can cause instability and aggregation. Using various excipients, a formulation can be created to ensure long-term stability and provide resilience against these stress factors.
Besides the different mechanisms that can lead to protein aggregation, antibody-drug products can be highly diverse, differing in hydrophobicity, charge distribution, and glycosylation. While polysorbate 20 and 80, poloxamer 188, and cyclodextrin can protect proteins from mechanical stress, it is difficult to reliably and confidently predict which excipient is the best choice for a particular protein. The same can be said for excipients stabilizing against thermal and thermodynamic factors. It is therefore essential to have access to a large portfolio of high-quality excipients to address a range of formulation challenges.
More detailed information on mechanisms involved in the formation of protein particles and why different classes of excipients are required to prevent protein aggregation is covered in our on-demand webinar “How to Prevent Protein Aggregation through Stabilizers and Surfactants”.
References
Para seguir leyendo, inicie sesión o cree una cuenta.
¿No tiene una cuenta?