Handling Inclusion Bodies in Recombinant Protein Expression
Recombinant proteins are most often expressed in the intracellular space, but expression can also be controlled so that the protein is secreted into the periplasmic space or out into the culture medium. While secretion is advantageous in terms of protein folding, solubility, and disulfide bonding, the yield is generally much higher when using intracellular expression.
However, recombinant protein accumulated intracellularly is frequently deposited in the form of inclusion bodies, insoluble aggregates of misfolded protein lacking biological activity. The recombinant protein is often the major component of the inclusion bodies. The preparation of inclusion bodies can therefore be a purification step of significant importance. The isolation of proteins from inclusion bodies, though, often leads to difficulties with refolding and usually does not give full recovery of biological activity. Table 10.1 summarizes the advantages and disadvantages of working with recombinant products expressed as inclusion bodies. Inclusion body formation frequently occurs when eukaryotic proteins are expressed in bacterial hosts.
If the protein is expressed as inclusion bodies, there are several options to consider: optimize as much as possible for soluble expression, accept the formation of inclusion bodies but develop strategies to solubilize and refold the protein, try another expression host, or modify the plasmid construct. Expression as inclusion bodies can allow expression of proteins that are toxic to the host cell.
Optimizing for soluble expression
The reasons for inclusion body formation are not well understood. However, it is well known that a reduced growth rate usually leads to more soluble expression and hence reduces the tendency to form inclusion bodies.
A few straightforward modifications to culture conditions, aimed at reducing the growth rate and/or the rate of expression, are thus worthwhile to consider for optimizing soluble expression. A drawback is that the overall yield of recombinant protein is also likely to decrease as a result.
A reduced growth rate can be achieved by lowering the growth temperature to between 20 °C and 30 °C.
For proteins that are expressed under the control of an inducible promoter, the rate of expression can also be reduced by altering the induction conditions:
- induce at lower cell densities (A600 = 0.5)
- induce for a shorter period of time
- induce using a lower concentration of the inducing agent (e.g., 0.1 mM IPTG)
Should these modifications prove insufficient, more comprehensive changes can be considered. These include the use of fusion tags, such as GST and maltose binding protein (MBP), which have been reported to enhance solubility (Reference: Esposito, D. and Chatterjee, D. K. Enhancement of soluble protein expression through the use of fusion tags. Current Opinion Biotech, 17, 353–358 (2006). Other options include coexpression with chaperonins or other folding-machinery components, and the use of an alternative host organism. A comprehensive description of procedures that increase soluble expression is outside the scope of this handbook.
Solubilizing inclusion bodies
If culture modifications do not significantly improve the yield of soluble tagged proteins, then common denaturants such as 4 to 6 M Gua-HCl, 4 to 8 M urea, detergents, alkaline pH (> 9), organic solvents, or N-laurylsarcosine can be used to solubilize inclusion bodies.
Success of affinity purification in the presence of denaturing agents will depend on the nature of the tagged protein. It is important to test the chosen denaturant with the target protein before introducing it into the solubilization strategy.
For each denaturant the success of solubilization will be affected by the presence and concentration of reducing agent, time, temperature, ionic strength, and the ratio of denaturant to protein. Refer to Table 10.2 for experimental starting points for solubilization of inclusion bodies. Solubilized proteins can often be purified at this stage by using a separation technique that is compatible with the presence of the denaturant. Purification and refolding can often be combined in the same purification step, for example, by chromatographic on-column refolding.
Many alternative solubilization protocols have been published (e.g., REFOLD database). Options include the use of SDS (10%), N-laurylsarcosine, or other detergents and extremes of pH.
Refolding of solubilized recombinant proteins
Following solubilization, proteins must be properly refolded to regain function. Denaturing agents must always be removed to allow refolding of the protein and formation of the correct intramolecular associations. Critical parameters during refolding include pH, presence of reducing reagents (often a mixture of reduced and oxidized forms of a weak reducing agent, e.g., glutathione, is used), the speed of denaturant removal, and the purity of the protein to be refolded. Table 10.3 compares conventional methods for refolding with on-column affinity purification and refolding.
Refolding usually requires extensive optimization. One should always consider other alternatives (as mentioned earlier), for example, optimizing expression parameters, making a new construct, or changing the expression host.
On-column refolding
Using a histidine-tagged protein enables the use of a simple, but efficient, purification and on-column refolding procedure that produces soluble protein exhibiting the desired biological activity. The protocol shown in Figure 10.1 has been used successfully for several different histidine-tagged proteins.

Figure 10.1.General scheme for the extraction, solubilization, and refolding of (histidine)6-tagged proteins produced as inclusion bodies in E. coli cells.
The strong binding of histidine-tagged proteins to immobilized divalent metal ions is not disrupted by high concentrations of chaotropic agents (such as urea or Gua-HCl). Consequently, (histidine)6-tagged proteins can be solubilized by chaotropic extraction and bound to Ni Sepharose. Removal of contaminating proteins and refolding by exchange to nondenaturing buffer conditions can be performed before elution of the protein from the column.
Once refolded, the protein may be purified further by any other chromatography technique as for any native protein (Chapter 8, Purification of protein A-tagged proteins) if a higher degree of purity is required.
Analysis of correctly folded proteins
Table 10.4 summarizes various techniques used to assess whether proteins are correctly folded.
Application
Purification and on-column refolding of an insoluble histidine-tagged protein from a 100 mL E. coli culture using HisTrap FF 1 mL with ÄKTAprime plus
This procedure uses a HisTrap FF 1 mL column but also can be used with a HisTrap HP 1 mL or a HisTrap FF crude 1 mL column.
Preparing the buffers
Use high-purity water and chemicals, and pass all buffers through a 0.45 µM filter before use.
Alternative binding buffers: 5 to 40 mM imidazole can be included in the binding buffer to reduce nonspecific binding of non-histidine-tagged proteins. The concentration of imidazole is protein dependent, and if the protein of interest elutes or does not bind at a certain imidazole concentration, reduce the concentration.
Disruption, wash, and isolation of inclusion bodies
- Resuspend the cell paste from 100 mL culture in 4 mL resuspension buffer.
- Disrupt cells with sonication on ice (e.g., 4 × 10 s).
- Centrifuge at high speed for 10 min at 4 °C.
- Remove supernatant and resuspend pellet in 3 mL of cold isolation buffer. Sonicate as above.
- Centrifuge at high speed for 10 min at 4 °C.
- Repeat steps 4 and 5.
At this stage the pellet material can be washed once in buffer lacking urea and stored frozen for later processing.
Solubilization and sample preparation
- Resuspend pellet in 5 mL of binding buffer.
- Stir for 30 to 60 min at room temperature.
- Centrifuge for 15 min at high speed, 4 °C.
- Remove any remaining particles by passing sample through a 0.45 µM filter.
The optimal concentration of β-mercaptoethanol (0 to 20 mM) must be determined experimentally for each individual protein.
If it has not been prepared as above, adjust the sample to the composition of binding buffer by diluting in binding buffer or by buffer exchange using a desalting column (Chapter 11, Desalting/Buffer Exchange and Concentration), then pass the sample through a 0.45 µM filter.
Preparing the system
If a linear gradient formation for refolding and elution is chosen, the use of a chromatography system is essential.
This example uses ÄKTAprime plus. Once the system is prepared, the remaining steps (under Selecting Application Template and starting the method) will be performed automatically.
- Place each inlet tubing from port A (8-port valve) in eluents as given above and the tubing from port B (2-port valve) in the elution buffer.
- Place the three brown waste tubings in waste.
- Connect the column between port 1 on the injection valve (7-port valve) and the UV flow cell.
- Fill the fraction collector rack with 18 mm tubes (minimum 40) and position the white plate on the fractionation arm against the first tube.
- Connect a sample loop large enough for your sample between port 2 and 6 on the injection valve. Use a syringe to manually fill the loop.
Note: If a Superloop™ is needed, additional information is supplied in the instructions for Superloop.
Selecting Application Template and starting the method
- Check the communication to PrimeView. At the lower right corner of the screen the text Controlled By: prime should be displayed.
- Use the arrow and OK buttons to move in the menu tree until you find On-Column Refolding HisTrap.

3. Enter the sample volume and press OK to start the template.

Figure 10.2.Theoretical gradient in On-column Refolding HisTrap Application Template

Figure 10.3.On-column refolding of a histidine-tagged protein.
Screening conditions for refolding using IMAC
MultiTrap 96-well plates are useful products for screening of IMAC refolding conditions (Table 10.5). In this example, solubilized, unfolded protein was dispensed in His MultiTrap FF wells. The proteins were incubated in various refolding buffers, and the extent of refolding was determined (Figure 10.4). Optimal buffers from similar screening experiments can be used for scale-up on-column refolding on HisTrap FF.
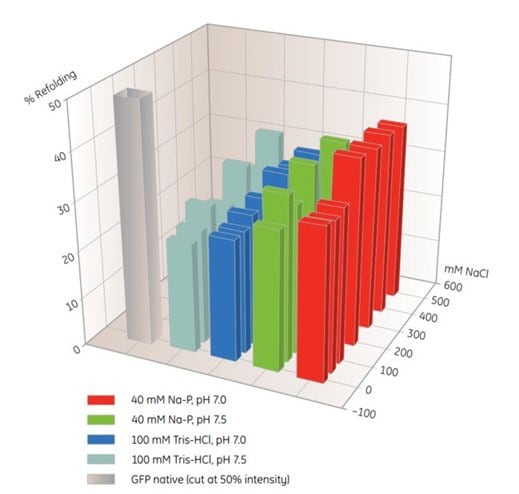
Figure. 10.4.Screening of IMAC refolding conditions for histidine-tagged GFP using His MultiTrap™ FF. This initial screen covered buffer substances, pH and salt concentrations. Data kindly provided by J. Buchner, M. Haslbeck and T. Dashivets, Munich Technical University, Germany.
To continue reading please sign in or create an account.
Don't Have An Account?