Introduction
Hashmi, Toste, Echavarren, and Haruta, among others, have fueled the advance of gold into the forefront of transition metal catalysis.1,2 Phosphine ligated gold(I) complexes have risen as powerful C–C, C–N, and C–O bond forming catalysts due to the ease with which they can activate C=C and C=C bonds thus allowing for unique rearrangements or reactions with various nucleophiles. Gold catalysis provides an excellent method to construct complex chemical architectures in a mild manner that would be difficult to achieve using other reaction paradigms. As illustrated below, the active catalysts are typically prepared by the addition of a silver activator to a gold halide precatalyst, although there are examples of isolable gold catalysts.

We are proud to offer a treasure trove of gold precatalysts and silver salts, as well as an extensive portfolio of unsaturated building blocks to accelerate your research success in this exciting field.
Advantages
- Catalysts readily activate alkenes, alkynes, and alkenes in a myriad of transformations
- Promote regio-, diastereo-, and enantioselective processes
- Catalysts are typically air- and water-stable and reactions can be performed in an “open flask”
- Exceptional functional group tolerance
- Market exclusivity for selected gold catalysts
Representative Applications
Rearrangement of Allylic Acetates
Marion et al. reported the catalyzed isomerization of allylic acetates. Using a gold complex with a N-heterocyclic carbine ligand, the researchers reported the first gold catalyzed allylic rearrangement. To illustrate the versatility of the catalyst, several allyl acetates were rearranged with excellent yields.

Figure 1.Rearrangement of Allylic Acetates
Isomerization of Allenyl Carbinol Esters
Buzas et al. reported the synthesis of a series of 1,3-butadien-2-ol from different allenes using a gold complex as catalyst. Butadiene building blocks are of interest for Diels-Alder reactions, [4+1] cycloaddition and asymmetric hydrogenation. The catalyst utilized in this reaction is a gold complex with XPhos as a ligand. With a low loading of 1mol%, the authors were able to synthesize a variety of butadiene with yields up to 100%.

Figure 2.Isomerization of Allenyl Carbinol Esters
Intramolecular [4+2] Cycloadditions of Arylalkynes or 1,3-Enynes with Alkenes
Echavarren and co-workers reported the synthesis of pycnantuquinones A derivatives, catalyzed by [4+2] cycloaddition of dienynes. Using a stable crystalline gold complex based on the Buchwald ligand, the researchers were able to synthesize a family of derivatives with good yields.
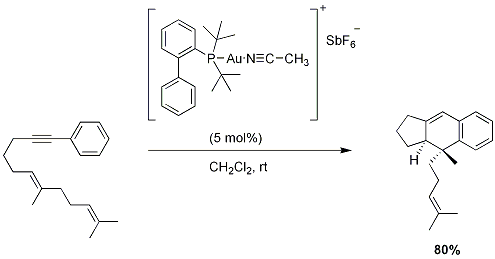
Figure 3.Cycloadditions of Arylalkynes
Cyclopropanation
The Toste group demonstrated that olefins undergo stereoselective cyclopropanation (cis) with propargyl esters in the presence of in situ generated Ph3PAuSbF6 and is thus a complement to the trans selectivity observed in transition metal catalyzed cyclopropanation of olefins using α-diazoacetates.

Figure 4.Cyclopropanation
Isomerization of 1,6-Enynes
Echavarren and others have studied a variety of gold catalyzed cyclizations of 1,6-enynes with or without the presence of nucleophiles. A variety of gold(I) catalysts are active, including Ph3PAuCH3, Ph3PAuSbF6, and Au[P(t-Bu)2(o-biphenyl)]SbF6 complexes. The reactions can tolerate heteroatoms located between the olefin and alkyne moieties.
Isomerization of 1,5-Enynes
Under gold(I) catalysis, 1,5-enynes of varying substitution patterns rearrange to give bicyclo[3.1.0]-hexenes in a high yielding, stereocontrolled fashion. For optically active substrates, the reaction can occur with efficient chirality transfer. The catalyst system utilizes Ph3PAuCl in combination with AgBF4, AgPF6, or AgSbF6 activators.
Isomerization of 1,4-Enynes (Rautenstrauch Rearrangement)
The Rautenstrauch rearrangement of 1,4-enynes provides an expeditious route to a diverse portfolio of functionalized cyclopentanones. Chiral 1-ethynyl-2-propenyl pivalates efficiently rearrange enantioselectively under mild conditions. Either Ph3PAuSbF6 or Ph3PAuOTf (both generated in situ) can be used, depending on the identity of the substrates.
Cyclization of ε-Acetylenic Carbonyls (Conia-Ene Reaction)
The non-catalyzed Conia-ene reaction provides access to methylenecyclopentanes without the need for deprotonation, however, the high temperatures required often result in diminished yields. Toste and co-workers reported a mild catalytic version of this reaction that proceeds under neutral conditions at ambient temperatures.
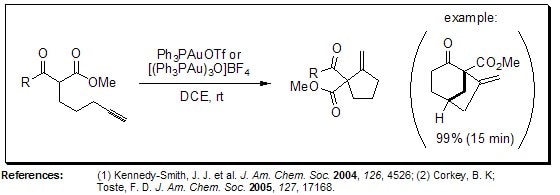
Figure 5.Conia-ene reaction
5-Endo-dig Carbocyclizations
While the gold(I)-catalyzed Conia-ene cycloisomerization is limited to terminal γ-alkynes, the related 5-endo-dig reaction allows for cyclization onto non-terminal δ-alkynes providing access to cyclopentene derivatives. This synthetic methodology can be applied to the preparation of simple bicyclic molecules, as well as in heterocycle synthesis (below) and halogenated cyclopentenes via alkynyl halide precursors.

Figure 6.Endo dig Carbocyclizations
Propargyl Claisen Rearrangement
The gold catalyst [(Ph3PAu)3O]BF4 provides access to a variety of homoallenic alcohols via a rapid two-step, one-pot sequence of a Claisen rearrangement of a propargyl vinyl ether followed by reduction of the aldehyde functionality. The reactions are generally high yielding and the robust catalyst system also shows superb ability to relay resident chirality into the allene products.

Figure 7.Propargyl Claisen Rearrangement
Stereoselective Synthesis of Dihydropyrans
Using gold(I) catalysis, 2-substituted dihydropyrans are readily prepared from propargyl vinyl ethers in a stereocontrolled fashion.
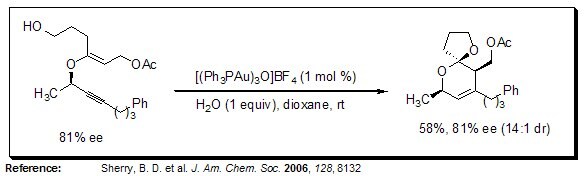
Figure 8.Stereoselective Synthesis of Dihydropyrans
Hydroamination of Alkenes and Alkynes
Widenhoefer and He have explored a variety of gold catalyzed hydroamination reactions with alkenes and alkynes, and both intra- and intermolecular variants were studied. Using either Au(I) or Au(III) catalysts, amines, pyrrolidines, imines, and indoles can be easily accessed.

Figure 9.Hydroamination of Alkenes and Alkynes
Hydrofunctionalization of Allenes with C, N, and O Nucleophiles
Vinylated tetrahydrofurans, tetrahydropyrans, pyrrolidines, and piperidines can be readily prepared from the corresponding heteroatom functionalized allenes using Au[P(t-Bu)2(o-biphenyl)]Cl and one of several silver activators. Alternatively, allene tethered indoles can be used in the preparation of carbazole derivatives.

Figure 10.Hydrofunctionalization of Allenes
Stereoselective Synthesis of Functionalized Dihydrofurans
Gagosz and co-workers have prepared a variety of 2,5-dihydrofurans via formation of an allene intermediate followed by cycloisomerization. The rapid reactions occur in the presence of Ph3PAuNTf2, and complete chirality transfer is observed.

Figure 11.Stereoselective Synthesis of Functionalized Dihydrofurans
Ring Expansions of Alkynylcycloalkanols
1-Alkynylcycloalkanols rapidly rearrange to the corresponding 2-alkylidenecycloalkanones in the presence of several gold catalysts. The high yielding reactions provide a single olefin isomer when internal alkynes are employed.

Figure 12.Ring Expansions of Alkynylcycloalkanols
Acetylenic Schmidt Reaction
In the presence of a gold catalyst, pyrroles of varying substitution patterns can be prepared by an intramolecular acetylenic Schmidt reaction of homopropargyl azides.
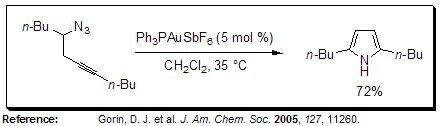
Figure 13.Acetylenic Schmidt Reaction
References
To continue reading please sign in or create an account.
Don't Have An Account?