Approaches to the Design of Lipid-based Nanocarriers for mRNA Delivery
Xing Duan, Xiangrong Song*
Department of Critical Care Medicine, Department of Clinical Pharmacy, Frontiers Science Center for Disease-related Molecular Network, State Key Laboratory of Biotherapy and Cancer Center, West China Hospital, Sichuan University, Chengdu, 610041, China.
Introduction
A variety of carriers, including lipid-based nanocarriers (LNPs), polymeric nanoparticles, cationic nanoemulsions (CNEs), and other delivery systems, have been synthesized to protect mRNA from degradation by widely present RNase and are crucial to the development of mRNA drugs. LNPs are the most prominent mRNA drug delivery systems due to their high efficiency and low toxicity. Two LNP-based mRNA vaccines, BNT162b2 (from Pfizer BioNTech) and mRNA-1273 (from Moderna), have been approved by the US FDA for controlling the SARS-CoV-2 pandemic.1 Generally, LNPs are composed of four components: cationic or ionizable lipids, neutral phospholipids, cholesterol, and polyethylene glycol-modified lipids. Cationic lipids were the first used to construct LNP delivery systems and have been widely used to deliver gene medicines, including DNA, siRNA, and plasmids et al. However, its development was restricted by its relatively low efficiency in delivering mRNA and certain toxic side effects. Therefore, correctly utilizing cationic lipids to develop efficient LNPs is a significant scientific problem that urgently needs to be studied.
Ionizable lipids are integral to the safety and efficacy of LNPs, and are crucial in determining mRNA loading, expression, and targeting.2 For example, the ionizable lipids used in Patisiran, BNT162b2, and mRNA-1273 are DLin-MC3-DMA (MC3), ALC-0315, and SM-102. However, it still needs to be clarified how the chemical structure of ionizable lipids affects the delivery efficiency of LNPs for mRNA. At the same time, developing efficient LNPs requires the meticulous design of formulations and the selection of appropriate routes of administration. Therefore, in this review, we explore how to use cationic lipids properly, develop highly efficient ionizable lipids, conduct innovative research on the prescription of LNPs, and choose the route of LNP administration.
Cationic Lipids for mRNA Delivery
Liposomes are considered the earliest lipid nanoparticles because they are composed of lipids and, in most cases, are nanoscale. Liposomes can effectively improve the water solubility of drugs and help most lipid-soluble drugs enter the clinic, such as doxorubicin liposomes and Epaxal. In nucleic acid drug delivery, cationic liposomes composed of cationic lipids with a constant positive charge can effectively carry negatively charged nucleic acids. Cationic lipids have been broadly used in mRNA delivery, including N-[1-(2,3-dioleoyloxy)propyl]-N,N,N-trimethylammonium chloride (DOTMA), 1,2-dioleoyloxy-3-trimethylammonium propane chloride (DOTAP)3, 1,2-stearoyl-3-trimethylammonium-propane (DSTAP), and 1,2-dimyristoyl-3-trimethylammonium-propane (DMTAP). Lipid nanoparticles constructed with these cationic lipids and other auxiliary materials can effectively deliver nucleic acid drugs within the body. However, with the emergence of ionizable lipids, nucleic acid delivery systems based on ionizable lipids show more efficient delivery performance than those based on cationic lipids. Therefore, the development of cationic lipids has entered a bottleneck period.
Selective Organ Targeting (SORT) Delivery System
Fortunately, researchers have found an effective way to use cationic lipids, the most prominent of which is the selective organ targeting (SORT) delivery theory. Cheng et al. replaced some auxiliary lipids with cationic lipids to construct an mRNA delivery system with a specific organ-targeting function. Adding cationic lipids could effectively regulate the zeta potential of LNPs and the type of protein adsorbed by LNPs during transport in the body, thus realizing organoselective LNPs. (Figure 1).4
Surface Modification and Functionalization of Cationic Liposomes
On the other hand, highly positively charged cationic nanovaccines may destroy blood cells and cause hemolysis. At the same time, they will be cleared by the reticuloendothelial system due to the absorption of excessive serum proteins, resulting in low efficiency of mRNA delivery in the body. To overcome this challenge, Song et al. prepared negatively charged mRNA vaccines by modifying natural anionic polymers onto the surface of cationic liposomes. This strategy effectively reduced the toxicity of cationic liposomes and achieved efficient mRNA delivery. Sodium alginate, hyaluronic acid, Dex-Aco, and other anionic polymers have been used to modify cationic liposomes, and this anionic coating strategy may have unique advantages in the development of cationic liposomes (Figure 2).5


Figure 1.Selective Organ Targeting (SORT) allows lipid nanoparticles (LNPs) to be systematically and predictably engineered to deliver mRNA into specific organs accurately. A) 5A2-SC8 SORT LNPs were formulated as indicated to make a series of LNPs with 0% to 100% SORT lipid (fraction of total lipids). Here, inclusion of a permanently cationic lipid (DOTAP) systematically shifted luciferase protein expression from the liver to spleen to lung as a function of DOTAP percentage. B) Quantification data demonstrated that SORT molecule percentage is the most crucial factor for tissue-specific delivery. Data were shown as mean±s.e.m. (n=4 biologically independent animals). C) Relative luciferase expression in each organ demonstrated that fractional expression could be predictably tuned (0.1 mg/kg Luc mRNA, IV, 6h). Data are shown as mean±s.e.m. (n=4 biologically independent animals). Reprinted with permission from reference 4, copyright 2020 Springer Nature.
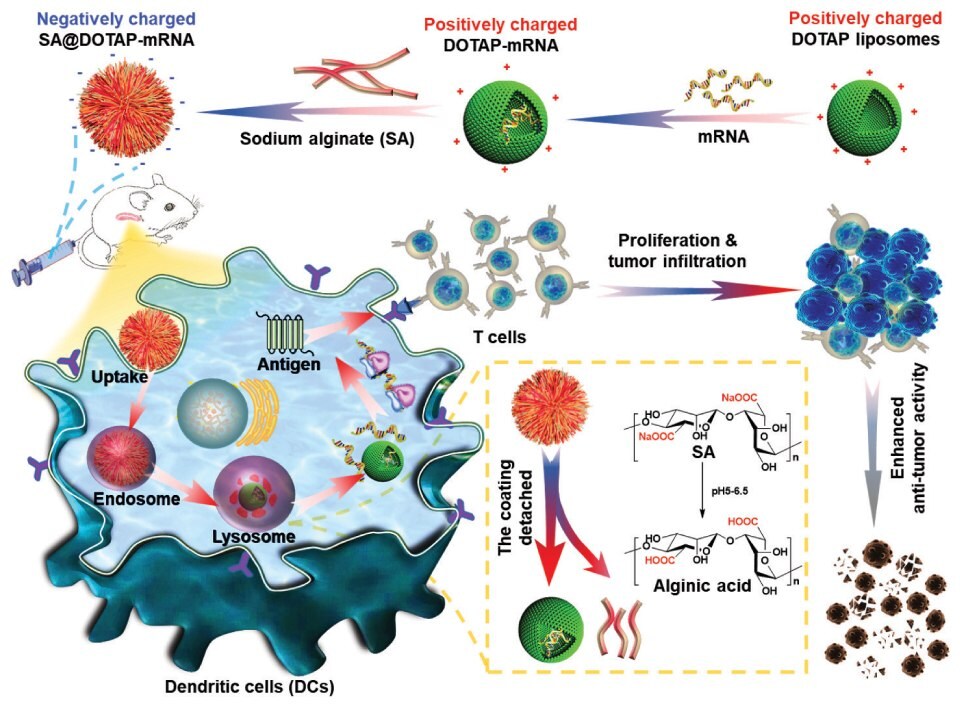
Figure 2.Negatively charged SA@DOTAP-mRNA was prepared by coating DOTAP-mRNA liposomes with SA, which contributed to the lysosome escape of the liposome/mRNA complex and induced a stronger immune response. Reprinted with permission from reference 5, copyright 2022 Elsevier.
Ionizable Lipids for mRNA Delivery
LNPs based on ionizable lipids have achieved promising therapeutic effects in clinical practice, and ionizable lipids, the core component of LNPs, play a decisive role in loading mRNA, protecting mRNA from RNase degradation, and controlling mRNA release. Clinically, the earliest ionizable lipid used to deliver nucleic acids was Dlin- MC3-DMA (MC3), which has been used to deliver siRNA clinically for treating transthyretin-mediated amyloidosis. In 2022, ionizable lipids ALC-0315 (Pfizer) and SM-102 (Moderna) were utilized in COVID-19 vaccine nanoparticles, becoming the only two mRNA vaccines in the world to be approved by the FDA for use against SARS-CoV-2. Currently, various mRNA drugs based on ionizable lipids are under clinical study.
The chemical structure of ionizable lipids plays a crucial role in nucleic acid delivery, so many preclinical studies have been conducted to reveal the effect of their chemical structure on nucleic acid delivery. Ionizable lipids are amphiphilic molecules that contain three domains: a polar head group, a hydrophobic tail region, and a linker between the two domains.
Polar Head Group of Ionizable Lipids
The head is composed of one or more protonatable N atoms. Although the three lipids currently on the market all contain only one protonatable N atom, it is unclear whether ionizable lipids whose heads contain a specific number of protonatable N atoms are more suitable for nucleic acid delivery. Song et al. designed a library of ionizable lipid compounds with a 4N4T structure. 4N4T ionizable lipids can be divided into hydrophilic centers and hydrophobic tails. The hydrophilic center consists of four tertiary amines (N) and four saturated hydrophobic chains (T). These 4N4T LNPs exhibit much higher mRNA translation efficiency than the approved SM 102 LNPs, and these 4N4T-based COVID-19 mRNA vaccines successfully trigger robust and durable humoral immune responses against SARS-CoV-2 and its variants, including Delta and Omicron. Importantly, 4N4T-based COVID-19 mRNA vaccines have higher RBD-specific IgG titers and neutralizing antibody titers than mRNA vaccines based on SM 102.6 Other ionizing lipids based on multi-N atoms include C12-200, 5A2-SC8, 306Oi10, TT3, and C14-4.7 Although, according to the literature data, these carriers have not been compared head-to-head with SM102 or ALC-0315, they may have the same mRNA delivery capacity as SM102 and ALC0315.
The Hydrophobic Tail Region of Ionizable Lipids
Dan Peer et al. developed a series of ionizable lipids with different tail structures based on the same head. They found that lipids with a branched chain and ester bonds were less effective than those with linoleic fatty acid chains. This could be attributed to a better endosomal escape due to the structural change of linoleic lipids.8 In another study, a small library of lipoids using 3,3′-diamino-N-methyldipropylamine was designed to react with 11 saturated alkyl acrylate tails varying in length from 6 to 18. Results found that the lipoid 306Oi10 with a one-carbon branch in the tail conferred a tenfold improvement over the lipoid 306O10 with a straight tail. Nanoparticles containing 306Oi10 ionize at endosomal pH 5.0, thereby improving mRNA delivery.9
Linker Between the Polar Head Group and Hydrophobic Tail
The head and tail ligand structure of ionized lipids play an essential role in controlling the stability of LNPs, organ targeting during transport in vivo, and the release rate of nucleic acid molecules. Virgil Percec et al. found that LNPs had more obvious targeting in the liver and spleen when the ligand structure was an ester bond and more specific lung targeting when the ligand structure was an amide bond.10 This was also confirmed by the research work of Qiaobing Xu et al. By comparing 306Oi10 series ionizable lipids with 306N12B ionizable lipids, we can be better convinced that the linking groups play a prominent role in regulating the organ selectivity of LNPs.11 In addition, biodegradable lipids can also be made of both ester and disulfide motifs. Cleavage of the disulfide bonds drives an intraparticle nucleophilic attack on the ester linker, accelerating their degradation.12
Although the three components of ionizable lipids have their roles, the whole is greater than the local addition. In developing efficient ionizable lipids, we should design from the perspective of the whole molecule and choose the appropriate combination.
Research and Development of Highly Efficient Ionizable Lipids
Ionizable lipids are the core components of LNPs, so their development has attracted much attention. According to the review in Nature Communications, ionizable lipids are divided into five categories. Unsaturated ionizable lipids (DLin-MC3-DMA, A6, OF-O2, A18-Iso5-2DC18), multi-tail ionizable lipids (98N12-5, C12-200, 9A1P9 and cKK-E12), ionizable polymer-lipids (7C1 and G0-C14), biodegradable ionizable lipids (L319, 304O13, OF-Deg-Lin, 306-O12B) and branched-tail ionizable lipids (306Oi10, FTT). Based on current research progress and clinical status, degradable backbones and increased branching/tails are two of the most favorable structural properties for the future development of ionizable lipids. SM-102 and ALC-0315 have shared features, including a tertiary amine, branched tails, and ester linkers. Moreover, both exhibit extended aliphatic branches, making them resemble multi-tail structures. In addition to safety and potency, next-generation ionizable lipids with additional functions, such as targeting and immune regulation, will play an important role in specific applications. Currently, more than half of the LNPs are ionizable lipids targeted to the liver, spleen, lung, heart, kidney, pancreas, and other organs. There are still huge opportunities for innovation to realize the broader application prospects of RNA therapy.13
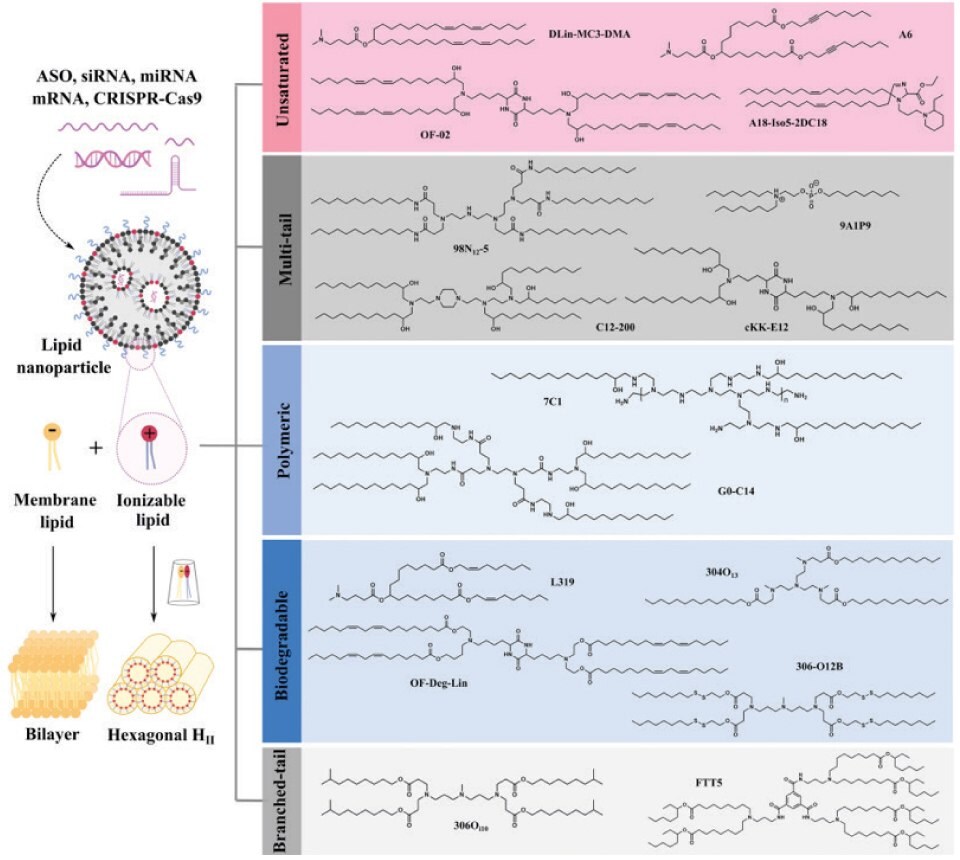
Figure 3.The cone-shaped ion pairs formed by anionic endosomal phospholipids and protonated ionizable lipids can disrupt the bilayer structure to promote endosomal escape. Based on their structural properties, RNA-delivering ionizable lipids can be categorized into unsaturated (containing unsaturated bonds), multi-tail (containing more than two tails), polymeric (containing polymer or dendrimer), biodegradable (containing biodegradable bonds) and branched-tail (containing branched tail) ones. Reprinted with permission from reference 13, copyright 2021 Springer Nature.
Prescription Design of LNPs
The formulations of commercially available LNPs are very similar. The formulations of LNPS consist of 35%-50% ionizable lipids, and the remaining 10%-20% are neutral lipids, 35-40% cholesterol, and 0.5%-2.5% PEG. In addition to ionizable lipids, other innovations are possible, such as replacing cholesterol with ß-sitosterol. ß-Sitosterol gives LNPs a polyhedral shape, which is conducive to endosome escape. The optimized LNPs showed uniform particle distribution, polyhedral morphology, and rapid stretched diffusion, which enhanced gene transfection.14 In addition, neutral phospholipids are replaced by cationic or anionic phospholipids to regulate the zeta potential of LNPs and achieve organ-selective delivery of LNPs.15 Other types of phospholipids and PGE are also being developed to improve the delivery capacity of LNPs. Figure 4 shows representative structures of LPN components.
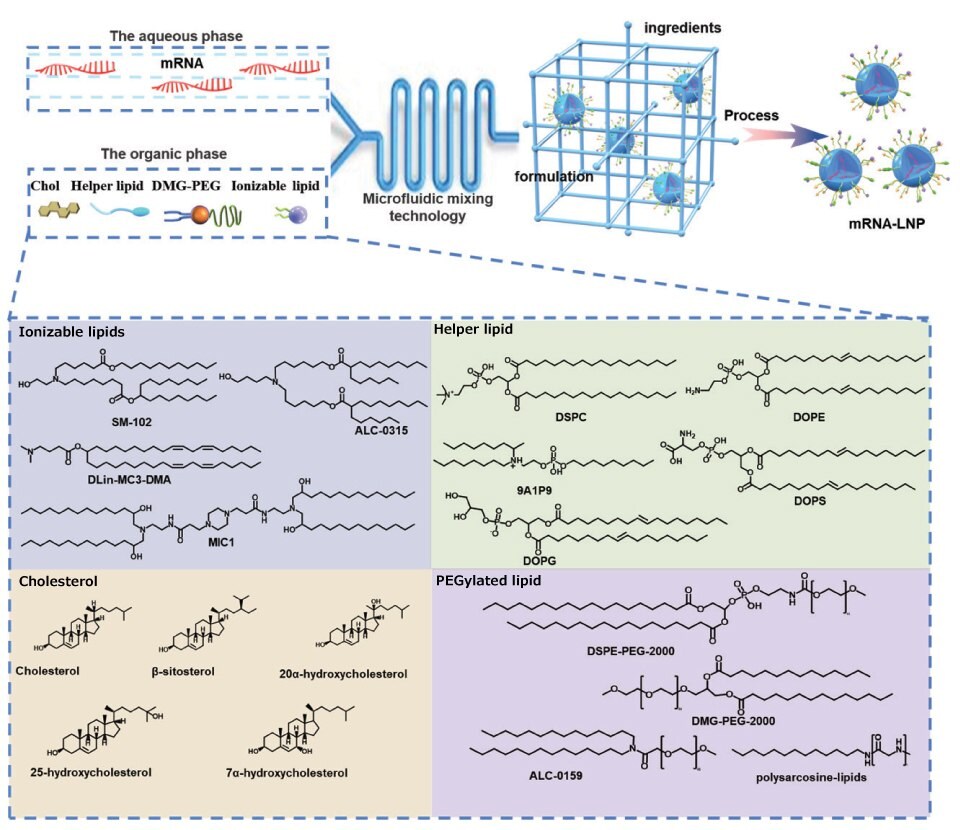
Figure 4.Representative structures of LNP components. LNP composition, prescription ratio, and preparation technology all have a crucial influence on the effect of LNPs. LNPs usually contain four components, namely, ionizable cationic lipids, phospholipids, cholesterol, and PEGylated lipids. They can self-assemble into nanosized LNPs via the ethanol dilution method. Here, representative compounds for each component are listed.
Administration Route of LNPs
Different modes of administration of LNPs will significantly affect the distribution of LNPs in organs, mRNA expression duration, and the types of cells that ingest LNPs, thus leading to different therapeutic effects. Intravenous and intramuscular injections of LNPs have been clinically applied, while other modes of administration are still under clinical and preclinical study. Yuhong Xu et al. injected LNPs through intramuscular, subcutaneous, and intravenous routes, and the results showed that LNPs in intravenous injection mainly accumulated in the liver, and the expression of luciferase in the liver peaked at 4 h and rapidly decreased within 48 h. LNPs injected intramuscularly may reside at the injection site, but gene expression is also evident in the liver. LNPs injected subcutaneously resided at the injection site and produced weaker mRNA expression.16 Kathryn A. Whitehead et al. selected 396Oi10, 200Oi10, and 514Oi10 to prepare three LNPs. Compared with intravenous injection (IV), intra-abdominal injection (IP) significantly enhanced the effectiveness and specificity of mRNA-targeted pancreatic delivery and helped to improve delivery and specificity.17 Also, based on the elaborate design of prescriptions, James E. Dahlman et al. constructed an LNP called Nebulized Lung Delivery 1 (NLD1) for further analysis. By comparing the relative sizes of different DLS peaks, the researchers found that NLD1 was more stable than 7C3, cKK-E12, and MC3 after nebulization. Finally, NLD1 was stable and well tolerated after nebulization, and the lung transfection efficiency was higher.18 Other drug delivery routes have also been used for LNP delivery. Niren Murthy et al. delivered LNPs into fetal mice by intrauterine injection and achieved effective transfection in the liver, heart, kidney, lung, gastrointestinal tract, and brain, with transfection levels exceeding 5%. This strategy is promising for the prevention and treatment of a variety of fetal diseases.19

Figure 5.Administration routes for LNP–mRNA formulations. LNPs can be treated by intravenous injection, intramuscular injection, subcutaneous injection, intradermal injection, and aspiration administration. Copyright Springer Nature Limited.
Perspectives
In the past decade, the development of LNPs has grown significantly. Currently, there are three commercially available LNPs and several clinical and preclinical studies related to LNPs. An increasing number of LNPs are expected to enter the market in the next few years. This paper summarizes the status quo of LNPs in recent years and some essential key points to guide future LNP research and development.
First, efficient delivery vectors are the key to the success of LNPs. Although cationic lipids may not be as good as ionizable lipids for mRNA delivery, they should not be abandoned. Surface modification, prescription optimization, and strategies of mixing with ionizable lipids may contribute to the development of cationic lipids. Second, when developing new lipid molecules, the three components of lipids should be taken into full consideration, and the chemical structure of the carrier should be adjusted for research and development to design an efficient, safe, and organ-selective carrier. Third, the design of prescriptions and the choice of administration route are also significant in the research and development of LNPs. Many auxiliary compounds may improve the stability of LNPs and the delivery efficiency of LNPs to mRNA. At the same time, the administration route of LNPs also has a crucial impact on their efficacy. Adjusting the prescription of LNPs must be addressed, as LNPs face different delivery barriers under different delivery routes.
Finally, each part of LNP research and development is essential, and the whole is greater than the sum of the parts. Researchers should properly design the chemical structure of the delivery vector, fine-tune the prescription composition of LNPs, and select the appropriate route of administration according to their own research and development purposes.
Acknowledgments
This study was supported by the National Key Research and Development Program of China (2021YFE0206600), the Sichuan Province Science and Technology Support Program (2021YFSY0008 and 2020YJ0238), the Translational Medicine Fund of West China Hospital (CGZH19002) and the 1.3.5 Project for Disciplines of excellence, West China Hospital, Sichuan University (ZYGD18020/ZYJC18006).
REFERENCES
Pour continuer à lire, veuillez vous connecter à votre compte ou en créer un.
Vous n'avez pas de compte ?