Analysis of Fluorescent Dyes Used for Labeling DNA in Microarray Experiments
Dr. Jens Sobek, Catharine Aquino, Dr. Ralph Schlapbach
Functional Genomics Center Zurich, Zurich, Switzerland
Cy3 and Cy5 are fluorescent dyes frequently used for microarray analysis. These two dyes have favorable properties, including relatively high fluorescence quantum yields and a minimal spectral overlap, making them suitable for multiplex detection applications. However, the Cy dyes and derivatives have low photostability compared to dyes that have been developed more recently.
The object of our study is to replace Cy5 dye in the detection of arrayed oligonucleotides with dyes that (1) are more stable and (2) give a higher fluorescence signal in microarray experiments. For this study we have developed a model system that allows us to investigate the fluorescence and stability properties of oligonucleotide-dye conjugates hybridized to the slide surface without laborious, time-consuming sample preparation. We reduced the complexity of the experiment by spotting only a few oligonucleotides and by hybridizing a single dye-labeled complementary oligonucleotide to each slide. Here we present preliminary results that compare the established blue indocarbocyanine dyes Cy5, DY-647, and Alexa Fluor® 647, to promising candidates Atto 633 and Atto 647N. The results of the complete study will be published elsewhere.1
Experimental Details
Materials
Three 71-mer oligonucleotides (Operon, Cologne, Germany) that share a common 13-mer binding region were used in this study. The common 13-mer region is located at the proximal 3′ end (71prox), near the center (71cent), or at the distal 5′ end (71dist) of the oligonucleotide. 13-mer oligonucleotide-dye conjugates (5′-dye-TGCCTGAAGCTAT; ON-Dye) that contain the sequence (ON) complementary to the binding region in the 71-mers were synthesized by Proligo (Hamburg, Germany) (Atto 633, Atto 647N), Microsynth (Balgach, Switzerland) (Cy5), Dyomics (Jena, Germany) (DY-647), and IBA (Göttingen, Germany) (Alexa Fluor® 647). All dyes except Cy5 were incorporated as the NHS ester via an aminohexyl linker in a separate reaction following oligonucleotide synthesis. For incorporation of Cy5, the phosphoramidite building block was used as received from Glen Research (Sterling, VA, USA). Water was deionized and filtered using a Milli-Q® Synthesis A10 purification system. All oligonucleotides were purified by HPLC. Sodium chloride (molecular biology grade), HEPES (N-[2- hydroxyethyl]piperazine-N`-[2-ethanesulfonic acid], >99.5%), trisodium citrate (>99%), hydrochloric acid (p.a.), and sodium dodecyl sulfate (>98%) were obtained from Sigma (Buchs, Switzerland).
Array Production
A sciFLEXARRAYER (Scienion, Berlin, Germany) and a Piezorray™ (Perkin Elmer, Downers Grove, IL, USA) non-contact dispensing instrument were used for microarray production. A dilution series (20, 10, 5, and 2.5 μM) of the three 71-mer oligonucleotides (71prox, 71cent, and 71dist) in 3× saline-sodium citrate buffer (SSC) were spotted in 15 replicates (1.5 nL per spot) on epoxysilane coated slides (Scienion, Berlin, Germany). Oligonucleotides were immobilized to the slide surface by overnight incubation in a humidity chamber at room temperature.2
Hybridization
Washing, blocking, hybridization, and drying steps were performed in an automated hybridization station (HS4800, Tecan, Salzburg, Austria), allowing 12 slides to be hybridized in parallel. 50 nM solutions of the 13-mer oligonucleotide-dye conjugates were hybridized to four replicate slides at 30 °C in HEPES/SSC/SDS for 2 hours. After hybridization, the slides were washed under non-stringent conditions at 23 °C sequentially with 2× SSC / 0.2% SDS, 0.2 × SSC / 0.2% SDS, and 0.2× SSC. The slides were then dried with nitrogen in the hybridization station to avoid air contact. After drying, the slides were quickly transferred to a plastic bag filled with nitrogen to reduce the time of air exposure to 10–15 seconds. Once in the bag, the slides were covered with blank glass slides to protect the dyes at the microarray surface from degradation by ozone and other oxidizing agents present in the air.
Determination of Dye Stability
To determine dye stability, the slides were scanned 12 times under a nitrogen atmosphere. Data provided by a nearby environmental measuring station (Stampfenbachplatz, Zurich) were used to estimate the atmospheric ozone concentration. For a correlation with these values, the concentration of atmospheric ozone in our laboratory was measured once using a spectrophotometer.3 We found that the ozone concentration in our laboratory was ~75% of the concentration measured at Stampfenbachplatz.4
Scanning and Data Analysis
All slides were scanned under identical conditions using a Tecan LS400 laser scanner. Arrays were protected by a cover glass and scanned with the hybridized side facing downwards. The scanner focus was set to the lower side of the slide and fluorescence was measured through the glass. Scans were evaluated using Array-Pro® Analyzer 4.5.1 software (Media Cybernetics, Silver Spring, MD, USA). Reported mean fluorescence intensity values are calculated from four replicate slides.
Absorption and Fluorescence Spectra
Absorption and fluorescence spectra were taken in 1× SSC using a Tecan Safire2™ spectrometer. Fluorescence was excited at 600 nm and detected at the surface of the solution (top reading mode).

Figure 1. Chemical structures of indocarbocyanines and carborhodamines dyes.

Results and Discussion
Molecular structures of the dyes evaluated are shown in Figure 1. The core chromophore structure is identical for the classical indocarbocyanine dyes Cy5, DY-647, and Alexa Fluor 647. The compounds differ by the types and location of alkyl groups and the number of sulfonic acid groups present, which affects the overall charge of the core (+1, -1, and -3, respectively, for the three dyes). Atto 633 and Atto 647N belong to the class of carborhodamine dyes, and both carry a charge of +1.
Absorbance and fluorescence spectra for all five dyes are shown in Figure 2. An analysis of the spectra will be given in a forthcoming publication.1

Figure 2. Absorption (A) and fluorescence (B) spectra of oligonucleotidedye conjugates (ON-Dye).
Fluorescence Intensities
A typical image of a slide hybridized with ON-Atto 647N is shown in Figure 3. The relative fluorescence intensity of each oligonucleotide-dye conjugate was measured after hybridization to the three 71-mer oligonucleotide probes using 15 replicate spots on four replicate slides, and the data for each conjugate was averaged (Figure 4).
Analysis of the fluorescence data shows a non-uniform pattern. The most intense signals were observed for the oligonucleotide-dye conjugates ON-Cy5 and ON-Atto 647N hybridized to 71dist (Figure 4A) and 71cent (Figure 4B). The maximal intensity of ON-Cy5 and ON-Atto 647N was found to be ~50% higher than for the other three conjugates. Additionally, there were large differences in signal intensity between the five ON-dyes especially when hybridized to 71dist, and these differences correlate with dye charge. The positively charged dyes (Cy5, Atto 633, and Atto 647N) produced more intense fluorescent signals than DY-647 (charge -1) and Alexa Fluor 647 (charge -3). All five oligonucleotide-dye conjugates have comparable fluorescent intensities when hybridized to 71prox (Figure 4C).
To investigate the effect of dye charge on signal, a second set of hybridizations were performed using 5×SSC/0.1% SDS buffer at 23 °C in the hybridization step. Under these less stringent conditions, no differences in fluorescence intensities for a given dye-labeled 13-mer were observed after hybridization to the three 71-mers (data not shown). The fluorescent intensities correlated with the dye charges, with Cy5 and Atto 647N conjugates again produced the highest intensities whereas those of the negatively charged dyes DY-647 and Alexa Fluor 647 dyes were up to 60% lower.
The data suggest that differences in fluorescence intensities at 30 °C result from a destabilization of the oligonucleotide hybrid caused by an interaction with the negative dye charges. Such hybrid destabilization was observed by Waggoner and others in 27-mer oligonucleotides multiply labeled with Cy3 at C-5 position of thymine,4 in 24-mers backbone-labeled with fluorescein and eosin,5 or a rhodamine attached to the 2-position of a nonamer.6 However, to the best of our knowledge, a destabilizing interaction caused by a labeling dye attached to one end of an oligonucleotide has not been reported previously.
Our results suggest that, for applications using short oligonucleotides, positively charged labeling dyes (e.g., Cy5, Atto 647N, Atto 633) are recommended to avoid the observed destabilizing effects.
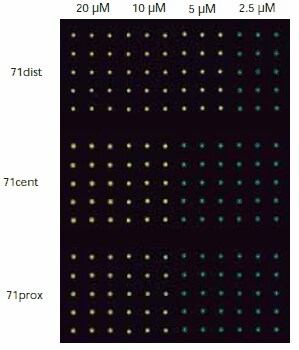
Figure 3. Fluorescence image measured with a confocal microscope. The spot-to-spot distance is 500 μM.

Figure 4. Fluorescence intensities of oligonucleotide-dye conjugates (ON-Dye) after hybridization to complementary 71-mer oligonucleotide (A, 71prox, B, 71cent, or C, 71dist) immobilized on an epoxysilane coated surface.
Investigation of Dye Stability
A limitation of many labeling dyes that absorb at long wavelengths, especially cyanine dyes including Cy5, DY-647, and Alexa Fluor 647, is an intrinsic low photostability and enhanced degradation by atmospheric ozone.7 Rapid dye degradation due to high ozone concentration during slide scanning may result in invalid data. In Zurich, ozone concentrations of 10–30 ppb and 10-75+ ppb were measured during winter and summer, respectively. A few minutes of exposure of unprotected slides at the highest ozone concentrations will result in a notable degradation of indocarbocyanine dyes.
To compare the dye stability, we performed two sets of experiments. First, to determine photostability hybridized slides were scanned 12 times. Since photodegradation strongly increases with increasing ozone concentration we scanned the slides under nitrogen using a protective cover glass (see Experimental Details). Second, an alternative batch of hybridized slides was stored under ambient laboratory conditions in the dark and exposed to air containing ozone concentrations of ~15 ppb for 30 minutes and 30 ppb for 150 minutes, respectively. Slides were scanned before and after this treatment under nitrogen.
Scanning the slides 12 times resulted in signal degradation between 3% and 9%, with Atto 647N demonstrating the least degradation (Figure 5). The most prominent signal intensity decrease was observed for Cy5 and Atto 633.

Figure 5. Dye degradation by irradiation. Hybridized slides were scanned 12 times. The ratio of fluorescence intensities of the first and last scan are plotted. To exclude the influence of ozone, slices were scanned under nitrogen.
Exposure of cyanine dyes to ozone had a tremendous effect on fluorescence intensity of dyes at surfaces (Figure 6). A 30 minute exposure of ON-Cy5 to a relatively low ozone concentration (15 ppb) resulted in a 30% loss in fluorescence intensity. Under these conditions, the sulfonated dyes (ON-DY-647 and ON-Alexa Fluor 647) are more stable. At a higher air ozone concentration (30 ppb) and with prolonged exposure (150 minutes), the signal intensity was reduced by 75% (Alexa Fluor 647), 79% (DY-647), and 89% (Cy5). The Atto dyes demonstrated only a minor loss of fluorescence intensity, with a reduction of 9% for Atto 647N, and of less than 1% for Atto 633. We even observed an initial increase in fluorescence intensity for the carborhodamine-based Atto dyes.

Figure 6. Influence of atmospheric ozone on fluorescence intensity. Hybridized slides were exposed in the dark to ozone concentrations of A) 15 ppb (30 μg/m3) for 30 minutes, and B) 25 ppb (50 μg/m3) for 150 minutes. Intensities were measured before and after exposure.
Conclusions
We measured the relative fluorescence intensities and the stability of five oligonucleotide-dye conjugates after hybridization to three 71-mer oligonucleotides immobilized on a microarray glass surface. We observed fluorescence intensities that correlate to the overall charge of the dye, with more negatively charged dyes producing lower fluorescent intensity. We attribute this to a destabilization of the hybrid by the dye. This information may be valuable when selecting a dye for use in applications requiring oligonucleotides with low melting temperature.
All dyes tested are sufficiently stable to repeated scanning at the appropriate excitation wavelength when protected from atmospheric ozone. Atto 633 and Atto 647N showed minor loss of fluorescence signal on exposure to ozone, making their use as labeling dyes in microarray applications optimal in this regard. We are aware that the results of this study do not indicate how successfully these dyes may be used in secondary DNA labeling reactions with aminoallyl substituted nucleotides and subsequent hybridization. Further work is in progress.
References
Pour continuer à lire, veuillez vous connecter à votre compte ou en créer un.
Vous n'avez pas de compte ?