Analysis of Persistent Organic Pollutants in Fish and Milk
Katherine K. Stenerson, Analytical Sciences Liaison
Overview of Sections:
Introduction
Persistent organic pollutants (POPs) are compounds that are not easily degraded by chemical, biological, or photolytic processes and thus persist in the environment for long periods of time. Many of these compounds were put into commercial use after World War II in manufacturing, agricultural, and other applications. In addition to those that were specifically produced for the intended use, POPs also resulted from various industrial processes and the combustion of certain materials. An example of the latter is dioxins, which can be produced from waste incineration and the manufacture of paper and pulp.1 As designated by the Stockholm Convention, the POPs list originally consisted of 12 chemicals, including organochlorine pesticides, polychlorinated biphenyls (PCBs), and polychlorinated dibenzo-p-dioxins (PCDDs)/polychlorinated dibenzofurans (PCDFs). New compounds have since been added and these include additional organochlorine compounds, several organobromine compounds, perfluorooctanoic acid (PFOA), perfluorooctanesulfonic acid (PFOS), and chlorinated naphthalenes, and paraffins. New compounds under consideration for inclusion on the official POPs list are methoxychlor, the flame retardant Dechlorane Plus and the UV absorber UV-238.2 In addition to the compound classes previously mentioned, polynuclear aromatic hydrocarbons (PAHs) are also considered as POPs because of their lipophilicity and continuous presence in the environment.3
Since POPs are lipophilic, they will bioaccumulate in the fatty tissues of living organisms. Moving up the food chain, their concentration increases as they pass from one organism to another, a process known as “biomagnification”.1 As a result, POPs can end up in animal-based foods such as meat and milk. Exposure to these compounds has been associated with different toxic effects for humans, including cancer, immunotoxicity, developmental issues, and reproductive effects. For this reason, regulations on the limits of exposure via diet have been developed by various countries.
Various approaches exist for the extraction and analysis of POPs. In this work, the Quick, Easy, Cheap, Effective, Rugged, Safe (QuEChERS) sample preparation method developed by Anastassiades and Lehotay,4 was applied to two different food commodities: fish and milk. Specifically, QuEChERS was applied to the extraction and cleanup of PAHs from raw salmon and PCBs from cow’s milk. Fatty foods such as fish and milk are challenging matrices in that fats often get coextracted with the target analytes, causing interference and/or sensitivity issues during analysis. Two zirconia-based sorbents, Supel™ QuE Z-Sep and Z-Sep +, were evaluated for the cleanup of fatty samples. In work published by Sapozhnikova and Lehotay on extraction and analysis of POPs in catfish, it was found that Z-Sep was effective in background removal while obtaining good recovery and reproducibility.5 Z-Sep is proprietary zirconia-coated silica. Zirconia, with its empty d-orbitals, acts as an electron acceptor (Lewis Acid) and thus will bind molecules with electron-donating groups (Lewis Base). This includes the phosphate moiety in phospholipids and the OH groups in sterols, mono, and diglycerides, and the COOH group in free fatty acids. In the case of Z-Sep +, the silica particle is functionalized with both zirconia and C18. The purpose of the C18 is to act in a synergistic way in retaining hydrophobic molecules such as triglycerides that will not be removed by the zirconia. Analysis of the PCBs and PAHs was conducted by gas chromatography (GC) using different modes of detection; an electron capture detector (ECD) for the PCBs and mass spectrometry (MS) for the PAHs. The GC capillary columns used for both applications were specially selected to provide the necessary selectivity, temperature, and speed requirements.
EXPERIMENTAL
Samples of farm-raised salmon and whole cow’s milk were obtained at a local grocery store. For recovery studies, salmon was spiked with a mixture of 27 different PAHs and milk with 20 PCB congeners representing those most commonly occurring and the WHO-listed coplanars. The spiking levels were 100 ng/g for PAHs in salmon and 20 ng/g for PCB congeners in milk. QuEChERS extractions were conducted as described in Tables 1 and 2. The extracts were then subjected to cleanup with different sorbents per Table 3. The specified volume of extract was added to a tube containing the cleanup sorbent. The tube was then shaken for 1 min and centrifuged at 3400 rpm for 3 min. The resulting supernatant was analyzed by either GC-MS in selected ion mode (salmon extract for PAHs) or GC-ECD (milk extract for PCBs). The GC conditions for each analysis are listed in Tables 4 and 5. GC columns were specially selected to provide the necessary selectivity, temperature, and speed requirements. Specifically:
- Salmon/PAH analysis: SPB-608, 20 m x 0.18 mm I.D., 0.18 µm (custom dimension) provided resolution of benzo [b], [j], [k] fluoranthene isomers and benzo [a] and [e] pyrene isomers.
- Milk/PCB analysis: The SLB-5ms, 20 m x 0.18 mm I.D., 0.18 µm provided a high maximum temperature for column “bake-out” of a heavy matrix, and with a hydrogen carrier, a faster analysis time than a standard 30 m column.
Quantitation of the PAHs in the salmon extracts was done against a 5-point calibration curve prepared in solvent (5, 10, 20, 30, 50 ng/mL) with internal standard added to each level at 20 ng/mL. An uncleaned salmon extract was injected 3X prior to running the calibration standards and sample extracts. This was done to "prime" the GC-MS system and stabilize the response for the heavier PAHs. A 20 ng/mL check standard was run after each set of spiked samples to check instrument response.
Quantitation of the PCB congeners in the milk extracts was done against a 5-point calibration curve prepared in solvent (1, 5, 10, 20, 30 ng/mL). A 10 ng/mL check standard was run after each set of spiked samples to check instrument response. If a response drop was indicated, samples were re-run with a new calibration.
RESULTS AND DISCUSSION
Background Removal
GC-MS analysis in full scan mode was performed before and after cleanup on both sets of extracts. The effect of the cleanup varied with the matrix. In the case of salmon, there was a significant reduction in overall background for all cleanups (Figure 1), while with the milk there was a reduction only in the matrix peaks eluting after 15 minutes. This is shown for Z-Sep cleanup in Figure 2. In the salmon extracts, cleanups containing PSA showed peaks eluting in the first portion of the run, several of which coeluted with PAHs. This required blank subtraction in order to accurately quantitate the spiked samples. These peaks were most likely from contaminants bleeding off the PSA material.

Figure 1.Comparison of salmon extracts before and after cleanup, GC-MS full scan (all are same Y-scale).

Figure 2.Cow’s milk extract before and after cleanup with Z-Sep, GC-MS full scan (both are same Y-scale).
For both matrices, the Z-Sep cleanup resulted in the lowest background. After cleanup, all PAHs were easily detected using GC-MS/SIM from the spiked salmon samples; as shown in Figure 3 for Z-Sep. In the case of the milk extracts, background removal was evaluated by GC-MS, however, PCB analysis was performed by GC-ECD (Figure 4). This was done because the selective response of the ECD did not provide an adequate response to the compounds comprising the sample matrix, thus a difference in the background could not be discerned between cleaned and uncleaned extracts. It was also noted in the milk extracts that the clean-ups reduced the level of heavy, late eluting matrix, but did not totally eliminate it. This required a longer run time to "bake" it off the GC column. The use of backflush on the GC instrument could be used to eliminate this.
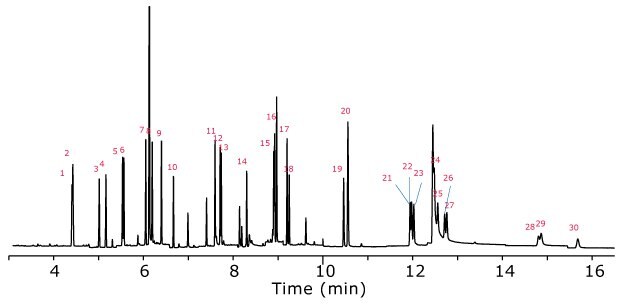
Figure 3.GC-MS/SIM analysis of PAHs extracted from salmon after Z-Sep cleanup. The spiking level was 100 ng/g. (Peak IDs Table 6)
Table 6. Peak ID PAH Compounds
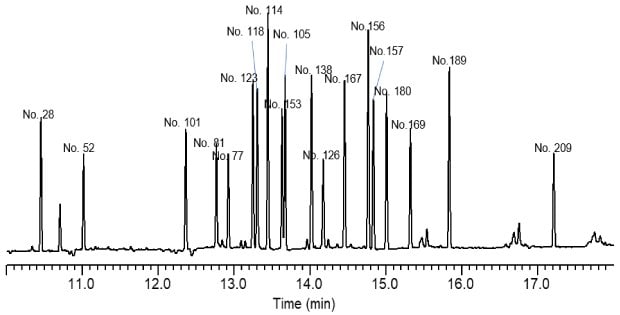
Figure 4.GC-ECD analysis of PCB congeners (Table 7) extracted from whole cow’s milk, after Z-Sep cleanup. Spiking level of 20 ng/g.
Table 7. Congener determined in elution order
Analyte Recoveries
Recoveries of the PAHs spiked into salmon are summarized in Figure 5 for the different cleanups. Three replicates of salmon extract were cleaned using each sorbent type, with the averages reflected in the graph. No PAHs were detected in the blank salmon samples. Repeatability for all cleanups was very good, with RSDs <10% for most PAHs and cleanups (with many <5% RSD). Overall, Z-Sep and Z-Sep+ exhibited the best recoveries. Between these two cleanups, Z-Sep+ extracts had recoveries of >80% for all analytes, while Z-Sep performed well for most PAHs (recovery >70%). A general trend of decreasing recovery with increasing molecular weight was noted for all cleanups. This could be due to the more limited solubility of heavier PAHs in the acetonitrile extraction solvent. A less polar solvent would most likely lessen this trend, however, it would undoubtedly co-extract more matrix which in turn may require more extensive cleanup and/or use of MS/MS for increased sensitivity and selectivity.
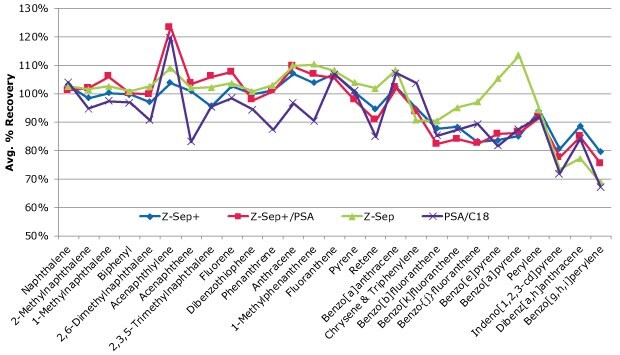
Figure 5.PAH recoveries from salmon, average of 3 spiked replicates (spiking level of 100 ng/g).
The recoveries of the PCBs from cow’s milk are shown in Figure 6. Overall, recoveries averaged in the range of 70-120% for a majority of the targeted congeners. It was noted that in general, recoveries declined with increasing molecular weight/degree of chlorination. Specifically, PCB congeners no. 157, 180, 169, 189, and 209 generally had recoveries <80%. Z-Sep showed a slight advantage over the other sorbents for recoveries of these congeners. Results between the different clean-up sorbents were comparable for the lighter congeners. Repeatability was very good for all clean-ups, with RSDs <10% for all but one congener/cleanup combination (congener no. 81 - Zep+/PSA cleanup).
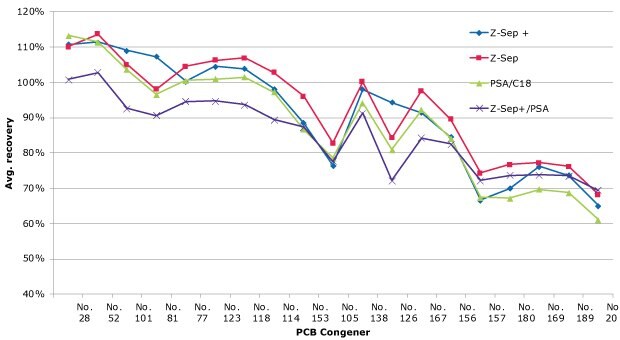
Figure 6.PCB recoveries from whole cow’s milk, average of 3 spiked replicates (spiking level of 20 ng/g).
CONCLUSION
QuEChERS extraction and cleanup were evaluated for the extraction of PAHs and PCBs from salmon and whole cow’s milk, respectively. For the cleanup step, different sorbents were evaluated. GC-MS data indicated that of the four cleanups, Z-Sep removed the most background in both the salmon and milk extracts. This agrees with the findings for catfish published by Sapozhnikova and Lehotay.5 For recoveries after cleanup, Z-Sep and Z-Sep+ yielded the best results for PAHs from salmon, while Z-Sep showed a slight advantage for PCB recovery from milk. In addition, the columns chosen for GC analysis offered advantages for these applications. The special dimension SPB®-608 provided resolution of PAH isomers and a faster analysis time. The SLB®-5ms provided a high maximum temperature for eluting heavy matrix, and the shorter length and narrow ID in combination with the hydrogen carrier provided for a faster analysis time while still achieving the required resolution.
Explore also the SLB®-ILPAH column on our ionic liquid GC column page, providing separation for difficult PAH isomer sets.
REFERENCES
To continue reading please sign in or create an account.
Don't Have An Account?