Click Chemistry in Drug Discovery
Perhaps no reaction in the click family has received more attention than the Cu(I)-catalyzed Huisgen 1,3-dipolar cycloaddition of terminal alkynes with organoazides to yield 1,4-disubstituted 1,2,3-triazoles (Scheme 1).1 True to a “good” click reaction, the reaction is reliable and high yielding, easy to perform, invariant to the presence of air or moisture, and tolerant of a wide range of functional groups. In many instances, water is the ideal reaction solvent, providing the best yields and highest rates. Typically, the cycloadducts are solids, eliminating the need for chromatographic purification. The 1,2,3-triazole ring is resistant to hydrolysis, oxidation, reduction, or other modes of cleavage. All of these properties make the Cu(I)-catalyzed azide-alkyne cyclization an important weapon in library development during the drug discovery process.2-4
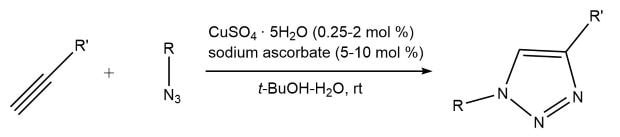
Scheme 1.Copper-catalyzed azide-alkyne cycloaddition reaction (CuAAC)
The use of a Cu(II) salt in the presence of an ascorbate reducing agent to form catalytically-active Cu(I) has been the method of choice for the preparative synthesis of 1,2,3-triazoles, but can be problematic in bioconjugation applications. A Cu(I) salt such as [Cu(CH3CN)4]PF6 may be used directly in the presence of the stabilizing ligand tris[(1-benzyl-1H-1,2,3-triazol-4-yl)methyl]amine, TBTA (Figure 1).8 TBTA has been shown to effectively enhance the copper-catalyzed cycloaddition without damaging biological scaffolds.5-7
![tris[(1-benzyl-1H-1,2,3-triazol-4-yl)methyl]amine, TBTA A chemical reaction scheme depicting the synthesis of a compound. On the left, two reactants are illustrated: a linear alkyne represented by "R'" and another molecule with an azide group (N3) attached to a variable group "R." The center of the image details the reaction conditions, specifying the use of copper sulfate pentahydrate (CuSO4·5H2O) at a concentration of 0.25-2 mol % and sodium ascorbate at 5-10 mol %. The reaction is conducted in a solvent mixture of t-butanol and water (t-BuOH-H2O) at room temperature (rt). On the right, the resulting product is shown, which features a six-membered ring containing two nitrogen atoms and the variable groups "R" and "R'."](/deepweb/assets/sigmaaldrich/marketing/global/images/technical-documents/articles/chemistry-and-synthesis/adc-and-bioconjugation/tbta-figure-1/tbta-figure-1.jpg)
Figure 1.Tris[(1-benzyl-1H-1,2,3-triazol-4-yl)methyl]amine, TBTA
Whereas the Cu(I)-catalyzed reaction provides access to 1,4- disubstituted triazoles, a transition metal variant allows for access to the complementary 1,5-isomer. Treatment of a terminal or internal alkyne with an azide in the presence of catalytic Cp*RuCl(PPh3)2 cleanly provides the cycloadduct in superb yield with complete control of regiospecificity (Scheme 2).4
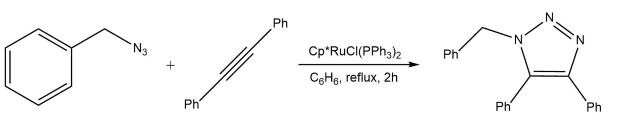
Scheme 2.Ruthenium-catalyzed one step azide-alkyne cycloaddition (RuAAC)
Of course, many organoazides are not commercially available. Carreira and co-workers recently reported the Co(II)-catalyzed hydroazidation of unactivated olefins with p-toluenesulfonyl azide (TsN3) to yield alkyl azides (Scheme 3).10-11 The catalyst is easily prepared in situ from Co(BF4)2·6H2O and a Schiff base ligand. Mono-, di-, and trisubstituted olefins are tolerated in the hydroazidation reaction, and complete Markovnikov selectivity is observed. Additionally, the reaction can be coupled to the Sharpless triazole cycloaddition to give the 1,4-triazole in a one-pot process.
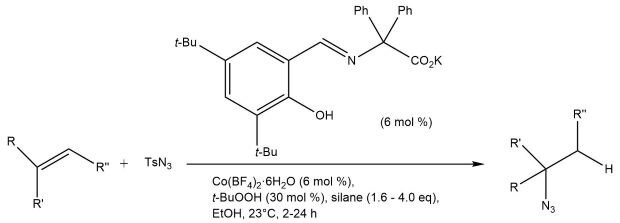
Scheme 3.Cobalt-catalyzed hydroazidation of alkenes.
View the full list of reagents, catalysts, and ligands for click chemistry applications.
References
To continue reading please sign in or create an account.
Don't Have An Account?