Robust Differentiation of Human iPSCs into Lineage-Specific Neurons and Glial Cells Utilizing Dual-SMAD Inhibition
Nick Asbrock, Christine Chen, Vi Chu
Introduction
The discovery that somatic cells could be converted into induced pluripotent stem (iPS) cells with the expression of four transcription factors (Oct-4, Klf-4, Sox-2, and c-Myc) has created an exciting new area of stem cell research1. Many human diseases that previously lacked a comprehensive in vitro cellular model can now be modeled using patient-derived iPS cells. Neurological diseases, such as Alzheimer’s disease, Parkinson’s disease, and autism were some of the first diseases modeled using an iPS cell approach. Highly enriched and scalable neural progenitor cells (NPCs) derived from iPS cells are attractive alternatives to tissue-isolated primary cells and immortalized neural stem cell lines for biomedical research. These iPS cell-derived NPCs offer an exciting opportunity to examine neuronal development and the commitment to specific neural cell types without ethical concerns associated with the use of human embryonic stem cells.
Dual SMAD inhibition is a well-established method to derive neural progenitor cells from both human ES and iPS cells2. This protocol uses two SMAD inhibitors, Noggin and SB431542, to drive the rapid differentiation of ES/iPS cells into a highly enriched population of NPCs2. Noggin acts as a BMP inhibitor and SB431542 inhibits the Lefty/Activin/TGFβ pathways by blocking the phosphorylation of ALK4, ALK5, and ALK7 receptors. In an effort to make a more defined and optimized neuronal differentiation protocol, Li and colleagues modified the original protocol to establish a completely small molecule-based differentiation method, which relies on three small molecules to inhibit GSK-3β (CHIR99021), TGFβ (SB431542), and Notch (compound E) signaling pathways, along with human LIF3. This new small molecule-based neural differentiation protocol increased neural differentiation kinetics and allowed the derivation of truly multipotent neural stem cells that respond to regional patterning cues specifying forebrain, midbrain, and hindbrain neural and glial subtypes.
Combining the above-described methodologies, we developed a new neuronal induction medium (NIM) and neuronal differentiation medium (NDM) to efficiently produce neural rosettes, expandable neural progenitors, end-stage neural (glutaminergic and dopaminergic) and glial subtypes (oligodendrocytes) from human ES/iPS cells. These media rely on a combination of commonly used neural supplements along with small molecule inhibitor combinations to achieve highly enriched population of multipotent NPCs. Many reports have demonstrated the propensity of the NPC’s differentiation toward a single lineage, either primarily neuronal or glial, but not both. Using our newly developed NIM and NDM media, iPS cells-derived NPC populations responded well to appropriate inductive patterning signals and could be directed towards both neural and glial cell lineages.

Figure 1.Workflow showing all steps in iPS cell generation and subsequent differentiation to neural and glial lineages. In as few as four steps, adult fibroblasts can be converted to neural and glial lineages using media formulations for modulating cell fate.
Methods
Neuronal differentiation of iPSCs using neural induction media
iPS cell-derived neural progenitor and differentiated neuronal cells were generated using the Human ES/iPS
Cell Neurogenesis kit (SCR603). Undifferentiated iPS cell colonies were seeded on to MEFs for 48 hours with 20% KOSR medium supplemented with FGF-2, 8 ng/mL (F0291). After 48 hours, growth medium was replaced by neural induction medium 1 (NIM1) for five days with medium changed every other day. On day six, cells were dissociated with non-enzymatic buffer (S-004-C) and plated on 0.5 mg/mL Matrigel® plates with neural induction medium 2 (NIM2) for an additional five days with medium changed every other day. After 10 days, neural progenitor cells were harvested with Accutase® reagent (A6964) and expanded with ENStem™-A medium (SCM004) supplemented with 20 ng/mL FGF-2 and 2 mM glutamine on the flask or plates coated with 0.1 to 0.2 mg/mL of Matrigel® at a density of 0.5 to 1 x 105 cells/cm2.
Differentiation of neural progenitor cells into neurons
Neural progenitors between P3 to P8 were harvested with Accutase® reagent (A6964) and NPCs were plated on poly- L-ornithine/laminin, 10 μg/mL each (P4957; CC095) at 10 to 20 thousand cells/cm2 with neuronal differentiation medium (SCM111) that was supplemented with 0.5 mM dibutyryl cAMP and 0.2 mM ascorbic acid phosphate. About 80% of the medium was refreshed every two to three days for a total of 9-14 days. Cells were fixed with 2% paraformaldehyde before immunofluorescent staining. Percentage of differentiated cells was determined by visual estimation.
Differentiation of neural progenitor cells into dopaminergic neurons
To derive dopaminergic neurons, the Dopaminergic Differentiation Growth Factor Sampler kit (SCR128) was incorporated into NIM-based neurogenesis protocol. 200 ng/mL of sonic hedgehog and 100 ng/mL FGF-8 were included in the NIM2 medium and cells were cultured for five days. Cells were then harvested with Accutase® reagent into single cells, and 3 x 104 NPCs were plated on poly-L-ornithine- and laminin-coated plates at 10 μg/mL each with neuronal differentiation medium containing 20 ng/mL BDNF and GDNF and 1 ng/mL of TGFβIII for 14 days. Tyrosine hydroxylase-positive cells representing dopaminergic neurons were characterized by immunofluorescent staining.
Differentiation of neural progenitor cells into oligodendrocytes
Oligoglial differentiation from iPS cells was performed by culturing neuronal progenitors at P1 in suspension culture with oligodendrocyte expansion medium (SCM107, basal medium with PDGF-AA, NT3, FGF2, T3) and retinoic acid for 14 days. The glial fate-primed neurospheres were then plated on poly-L-ornithine- and laminin-coated plated at 10 μg/mL each to further mature with Human OPC Spontaneous Differentiation Media (SCM106) for 14 days, with medium changes every two to three days.
Region-specific patterning of neuronal progenitor cells
iPS cells were induced to the neuronal lineages as described in the NIM user guide. On day five, cells were cultured for 10 more days in NIM2 (for spontaneous differentiation), NIM2 containing 200 ng/mL sonic hedgehog and 100 ng/mL FGF8 (SHH/FGF8; to prime cells for midbrain fate), or 200 ng/mL sonic hedgehog and 1 μM retinoic acid (SHH/RA; to prime cells for hindbrain fate). After 10 days, cells were harvested and the gene expression pattern was analyzed by qRT-PCR. GAPDH was used to normalize gene expression between samples. Fold changes in gene expression were calculated based on ΔCt values (normalized to GAPDH) and normalized to the spontaneous sample (spontaneous, n = 2).
Results
Over 80% Pax6-positive, polarized neural rosettes were generated from Oct-4-positive human iPS cells using NIM1 and NIM2 media after 10 days of differentiation. Pax6 expression increased in as little as 5 days, while mature neural rosettes structures, expressing both Pax6 and N-cadherin were observed after 10 days of culture. Neural rosettes could be dissociated and expanded up to 10 passages on Matrigel®-coated cultureware in ENStem™-A expansion medium, while maintaining a high level of Sox-2, Nestin, and N-cadherin expression.
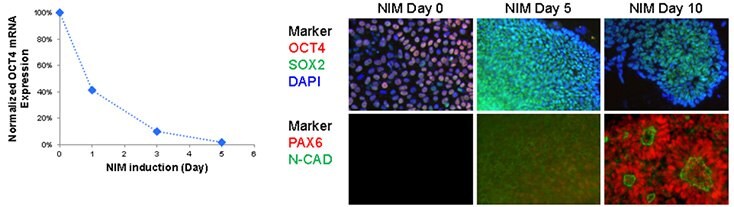
Figure 2.Neuronal differentiation of iPSCs A) Rapid down regulation of pluripotent maker, OCT4, in NIM protocol. Oct4 expression was drastically down regulated as early as 24 hour post neural induction. B) Up-regulation of neural makers in NIM protocol. Oct4 expression diminished after 5 days of NIM while Sox2 expression showed moderate increase during neural induction time course. Neural marker, PAX6 and N-cadherin are up-regulated after 10 days, forming polarized neural rosettes structures.

Figure 3.Characterization of iPSC derived neural progenitor cells. Passage 3 NPCs derived from iPS cells using NIM display typical neural progenitor morphologies (A) and were stained for NPC markers, Sox-2, N-cadherin (B), Pax6 and Nestin (C). These cells could be expanded for 10 passages while maintaining Nestin and Sox-2 expression.
iPS cell-derived neural progenitors were further differentiated into terminally differentiated neurons using Neuronal Differentiation Medium (NDM). Over 70% of differentiated cells were positive for TUJ1 and MAP2, markers of mature neurons, while less than 20% of the differentiated cells were positive for GFAP, a marker of astrocytes. Terminally differentiated neuronal cells were preferentially glutaminergic (VGlut+) rather than GABAergic neurons (GAD65 -) using NDM.

Figure 4.Neuronal differentiation of iPSC derived NPCs. After 9 days of differentiation, cells showed extended, elaborate neurite networks. The majority of the differentiated cells expressed neuronal markers, TUJ1 and MAP2 (A) with some cells differentiating to GFAP+ astrocytes. (B). Terminally differentiated cells were preferentially glutaminergic (C) rather than GABAergic neurons (D).
Both ES and iPS cell-derived neural progenitors can be directed to differentiate into tyrosine hydroxylase-positive dopaminergic neurons using NDM and a combination of growth factors (SCR128; SHH, FGF8, BDNF, GDNF, and TGFβIII). 10-30% of the differentiated culture population was tyrosine hydroxylase-positive after 14 days of differentiation.
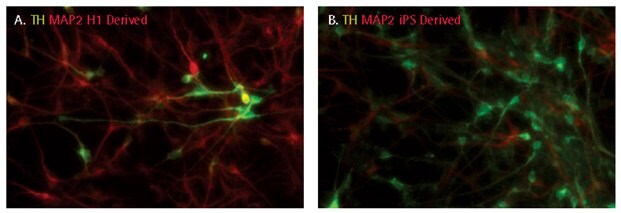
Figure 5.Dopaminergic differentiation of iPSC derived NPCs. After 14 days of differentiation, dopaminergic neurons were characterized by following the expression of tyrosine hydroxylase (TH). 10-30% cells were positive for tyrosine hydroxylase.
Human iPS cell-derived NPCs could efficiently differentiate into glial lineage using an proprietary oligodendrocyte differentiation protocol. The differentiated population was >80% positive for oligodendrocyte markers A2B5, O4, and Sox10, 10% GFAP positive, and 30% positive for GalC.

Figure 6.Oligodendrocyte differentiation of iPS cell derived NPCs. Cells were cultured as neurospheres in oligodendrocyte expansion medium and small molecules for 14 days before plating on poly-L-ornithine- and laminin-coated plates for immunocytochemical analyses for A2B5 (A), O4 (B), Sox10 (C), TUJ1 and GFAP (D), NG2, GalC (E), Sox-2 and Nestin (F) . Over 80% cells were A2B5-, O4- or Sox10-positive, 10% GFAP-positive, and 30% positive for GalC.
Human iPS cell-derived NPCs could be patterned into midbrain, forebrain or hindbrain neurons using NDM and specific patterning inducers (SHH/FGF8 or SHH/retinoic acid). Successful patterning was determined by using qRTPCR to measure the expression of region-specific markers.

Figure 7.Region-specific patterning of NPC neuronal differentiation. STEMCAA™-derived iPS cells were induced to the neural lineages as described. At day 5, cells were cultured in either NIM2 (spontaneous), or NIM2 with 200 ng/mL sonic hedgehog, 100 ng/mL FGF8 (SHH/FGF8), or 200 ng/mL sonic hedgehog, 1μM retinoic acid (SHH/RA) for 10 days to prime cells for midbrain and hindbrain fate, respectively. After 10 days, cells were harvested and the gene expression pattern was analyzed by qRT-PCR.
Conclusions
We have developed an efficient new neural induction and differentiation medium to generate highly expandable and multipotent neural progenitors and end-stage neuronal and glial cells from iPS cells. These media use established small molecule inhibitors and neural media supplements to produce rapid differentiation kinetics and efficiencies. We show that iPS cell-derived NPCs can be expanded for up to 10 passages while maintaining proper growth kinetics and marker expression. These NPCs are multipotent, differentiating into neurons, astrocytes, and oligodendrocytes depending on the environmental cues introduced into the culture conditions. Specific neural subtypes, such as TH+ dopaminergic and VGlut+ glutaminergic neurons could also be generated from the NPCs using commonly used growth factor inducers. These media provide a complete solution for researchers looking to model neurological diseases, such as Alzheimer’s disease, Parkinson’s disease and autism, using an iPS cell model system.
Materials
References
Pour continuer à lire, veuillez vous connecter à votre compte ou en créer un.
Vous n'avez pas de compte ?